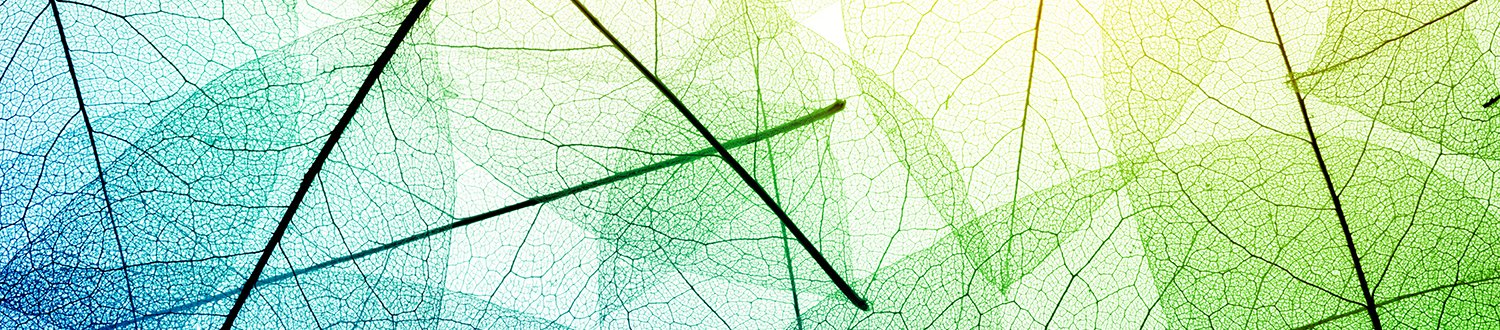
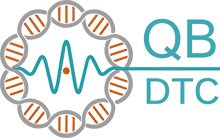
The role of decoherence and noise in biological systems
Noise is an inherent part of science, often referring to random fluctuations affecting data. It is usually considered to be a hindrance to be overcome as efficiently as possible. However, by understanding the way noise manifests, we can use it to improve efficiency of systems - for example, by noise-assisted transport.
Overview
In quantum mechanics noise is usually ignored so that the system of interest is simpler and the equations associated with it are easier to solve. But quantum mechanics is still applicable even in noisy environments, where the system of interest is coupled to a heat bath, which we refer to as an open quantum system. This is vital when we start looking at biological systems which are warm and messy and therefore noisy. A question we are asking is, could biological systems be using their noisy environments to aid processes like photosynthesis. There is evidence to suggest that this may be the case [1].
In molecular biology, it is often observed that there is some variance in the expression of particular mRNA and respective proteins between individual isogenic cells in a homogenous population of cells. This is called noise, and is measured as the coefficient of variation (CV):
CV = σ/μ
There are two components of noise: extrinsic and intrinsic[5]. Extrinsic noise can be thought of as failures in ensuring homogeneity, the development of micro-environments, fluctuations of conditions, and other uncontrolled factors. Intrinsic noise, however, is caused by the inherent randomness inside cells. Stochasticity is the property of, for example, transcriptional gene expression. Either the gene for protein ‘A’ is converted into RNA or it isn’t. The system is either on or off. Cells rely on the semi-random (Brownian) movements of molecules to cause reactions and interactions, however, which means that the activation of gene ‘A’ can be modelled as a probability. The observation that important biological mechanisms are governed by rates set by probability is an intrinsic source of noise.
Current projects
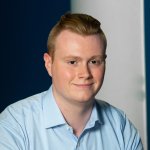
Exploring the role of gene expression noise on quantum decoherence in cellular systems
Michael Clarke-Whittet
Postgraduate researcher
Project description
Noise, defined as the standard deviation normalised to the mean of any set of observations, is a fundamental challenge in quantitative models of molecular biology. Michael will focus on characterising noise in a model organism and connecting observed noise to a novel cause of noise, post-transcriptional regulation. The 3’ untranslated region (UTR) of mRNA transcripts has been highlighted in previous work as a possible region for translational auto-regulation by corresponding RNA-binding proteins[4].
He will generate next-generation mNeonGreen-tagged strains in the model organism Saccharomyces cerevisiae. The strains will improve on previous strains by preserving the endogenous 3’ UTRs while allowing sensitive quantification of autoregulatory RNA-binding proteins. Quantification will be done using high-throughput flow cytometry, to provide powerful and reliable results. This basic research will give insights into the central dogma of biology and constitutes a missing link in modern models of gene expression for biomedical research and synthetic bio-syntheses which focus on transcriptional noise but not yet translation.
Supervisors
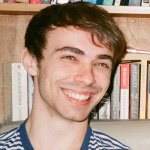
Non-Markovian dynamics in biomolecular complexes
Dr Adam Burgess
Postgraduate researcher
Project description
The recent discovery of long-lived coherences in complex biological complexes has raised a fundamental question of how the fluctuations (noise) present in the natural biological environment can possibly allow the preservation of quantum coherence. Indeed, it has been suggested that not only the noise fails to produce decoherence, it may even be the case that the fluctuations are a crucial ingredient in the maintenance of the coherence.
Supervisors
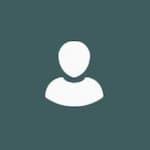
Vibrational coupling and non-adiabatic energy transfer in enzyme catalysis
George Ferguson
Postgraduate researcher
Project description
We seek to deepen our understanding of enzyme catalysis by assessing the role of quantum mechanics, and non-adiabatic energy transfer in these fundamental biological processes and shed light on one of the most fascinating and long-standing problems in biochemistry.
Supervisors
- Primary supervisor: Dr Marco Sacchi
- Co-supervisor: Dr Brendan Howlin.
Research team
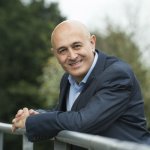
Professor Jim Al-Khalili
Distinguished Professor Emeritus in Physics
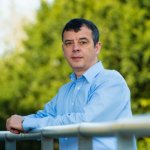
Professor Marian Florescu
Professor of Physics
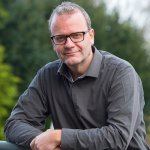
Professor Andre Gerber
Professor of RNA Biology
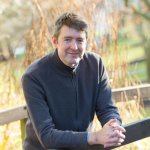
Professor Benedict Murdin
Professor of Physics, Head of the Photonics and Quantum Sciences Group
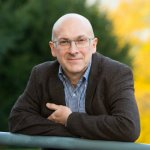
Dr Andrea Rocco
Associate Professor (Reader) in Physics and Mathematical Biology
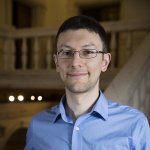
Dr Marco Sacchi
Associate Professor and Royal Society University Research Fellow in Physical and Computational Chemistry, Theme Leader in Sustainable Energy and Materials Research
References
[1] Caruso F, Chin AW, Datta A, Huelga SF, Plenio MB. Highly efficient energy excitation transfer in light-harvesting complexes: The fundamental role of noise-assisted transport. The Journal of Chemical Physics. 2009 Sep 14;131(10):09B612.
[2] Caldeira AO, Leggett AJ. Path integral approach to quantum Brownian motion. Physica A: Statistical mechanics and its Applications. 1983 Sep 1;121(3):587-616.
[3] Strunz WT, Yu T. Convolutionless non-Markovian master equations and quantum trajectories: Brownian motion. Physical Review A. 2004 May 24;69(5):052115.
[4] Mittal N, Scherrer T, Gerber AP, Janga SC. Interplay between posttranscriptional and posttranslational interactions of RNA-binding proteins. J Mol Biol. 2011;409(3):466–79.
[5] Elowitz MB, Levine AJ, Siggia ED, Swain PS. Stochastic gene expression in a single cell. Science. 2002 Aug 16;297(5584):1183–6.