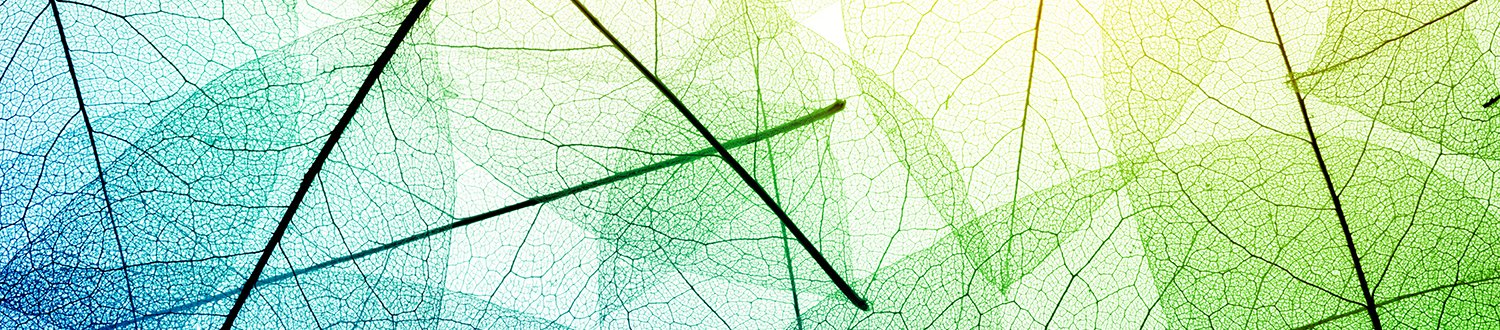
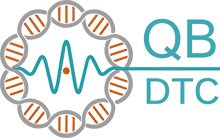
Quantum tunnelling in DNA
Tunnelling is a quantum phenomenon in which a particle is able to access a classically forbidden region. This often manifests as a “hopping” motion in which it appears that a particle has overcome an energy barrier which is greater than its kinetic energy. Studying quantum systems allows us to determine tunnelling rates and decide whether it is likely to happen.
Overview
In 1964, it was proposed by Per-Olov Löwdin [1] that quantum tunnelling can occur within the hydrogen bonds joining Watson-Crick nucleotide pairs. Since then, quantum tunneling has been explored as a mechanism at work in enzyme catalysis [2], olfaction [3], and other biological processes. Here at the Leverhulme Quantum Biology Doctoral Training Centre we use open quantum systems and density functional theory amongst other theoretical tools to make predictions about tunneling rates, and have experiments running in parallel to try and detect tunneling in the lab.
Current projects
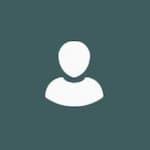
Measurement of proton position in DNA
Nermin Ener
Postgraduate researcher
Project description
Mutations are a cause of cancer and genetic disease as well as being the driver of drug-resistance in cancer and infectious disease. Watson and Crick proposed that these spontaneous mutations arise through tautomerization of DNA bases, involving the tunnelling of protons. However, despite a great deal of theoretical support for their model, experimental evidence is scanty, mostly because of difficulties in mapping proton position in the DNA double helix.
This project will bring together expertise in quantum biology and chemistry available at the University of Surrey with neutron crystallographic methods at the Institut Laue-Langevin (ILL) in Grenoble. The aim of this highly innovate, cutting-edge project will be to directly determine the protonation states in each of three major forms of DNA, A, B and Z, and to model these in the context of hydration-driven stereochemical pathways that are known to occur between them.
Particular attention will be focused on changes in protonation in these different forms and possible implication of tunnelling in mediating such shifts that may be a cause of mutation. This project will generate new data that will inform ongoing QB-DTC projects investigating tunnelling in DNA.
Supervisors
Modelling tunnelling in DNA base pairs
The 1964 work by Löwdin [1] proposes that there is a non-zero probability that the protons in hydrogen bonds holding together the two strands of DNA in the Watson-Crick model [4] can tunnel from one nucleotide to the other, dramatically altering the bond length. This spontaneous position shift transforms a base pair form the canonical to the tautomeric form. This causes an error in the genetic code, and accumulated errors of this kind could be responsible for mutation, ageing, and - in extreme cases - tumour formation. The work at the Leverhulme Centre builds on previous work carried out at Surrey [5].
In order to model this system, an open quantum systems [6] approach which uses the Caldeira-Leggett master equation [7] to describe the interaction of the proton in the hydrogen bond, the backbone of the DNA structure, and the surrounding cellular aqueous environment. Density functional theory is used to calculate the shape of the potential due to the DNA backbone. After solving the master equation, we explore the sensitivity of tunnelling rates due to the form of the environmental interaction, the asymmetry of the backbone potential, and the "memory" retained by the environment.
Although working closely together, Sapphire will focus on the mathematical behaviour described by the Caldeira-Leggett master equation and Louie will focus on the chemical properties of the DNA structure.
Experimental detection of tunnelling via mutation
Inspired by the experiments that demonstrated the importance of proton tunnelling in enzyme catalysis [8], Antonio and Virginia are currently investigating whether a similar kinetic isotope effect can alter the spontaneous mutation rate. If quantum tunnelling is involved in the transition of a DNA base from the common to its rare tautomeric form, then replacing a hydrogen nucleus (a single proton) with a deuterium nucleus (consisting of a proton and a neutron) should slow the transition rate since quantum tunnelling will be highly sensitive to doubling the mass of the particle trying to tunnel. By using deuterated water (D2O) as solvent rather than H2O and employing advanced experimental techniques from molecular biology, they aim to explore a potential relation between proton tunnelling and mutations.
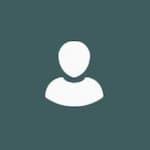
Virginia Tsiouri
Postgraduate researcher
Research team
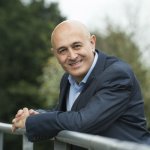
Professor Jim Al-Khalili
Distinguished Professor Emeritus in Physics
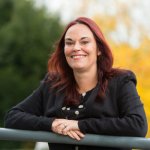
Dr Suzie Hingley-Wilson
Lecturer in Bacteriology
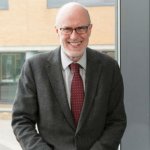
Professor Johnjoe McFadden
Professor of Molecular Genetics, Associate Dean (International)
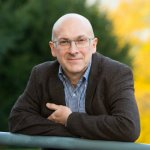
Dr Andrea Rocco
Associate Professor in Physics and Mathematical Biology
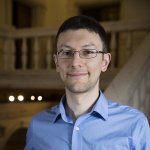
Dr Marco Sacchi
Associate Professor and Royal Society University Research Fellow in Physical and Computational Chemistry, Theme Leader in Sustainable Energy and Materials Research
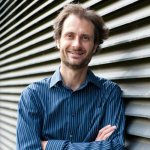
Dr Daniel Whelligan
Senior Lecturer in Organic/Medicinal Chemistry
References
[1] Löwdin PO. Proton tunneling in DNA and its biological implications. Reviews of Modern Physics. 1963 Jul 1;35(3):724.
[2] Devault D, Parkes JH, Chance B. Electron tunnelling in cytochromes. Nature. 1967 Aug;215(5101):642.
[3] Bittner ER, Madalan A, Czader A, Roman G. Quantum origins of molecular recognition and olfaction in Drosophila. The Journal of Chemical Physics. 2012 Dec 14;137(22):22A551.
[4] Watson JD, Crick FH. Molecular structure of nucleic acids. Nature. 1953 Apr 25;171(4356):737-8.
[5] Godbeer AD, Al-Khalili JS, Stevenson PD. Modelling proton tunnelling in the adenine–thymine base pair. Physical Chemistry Chemical Physics. 2015;17(19):13034-44.
[6] Breuer HP, Petruccione F. The theory of open quantum systems. Oxford University Press on Demand; 2002.
[7] Caldeira AO, Leggett AJ. Path integral approach to quantum Brownian motion. Physica A: Statistical mechanics and its Applications. 1983 Sep 1;121(3):587-616.
[8] Klinman JP, Offenbacher AR. Understanding biological hydrogen transfer through the lens of temperature dependent kinetic isotope effects. Accounts of chemical research. 2018 Aug 28;51(9):1966-74.