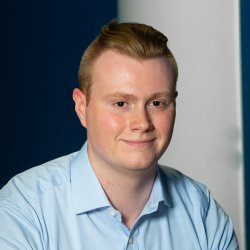
Dr Michael Clarke-Whittet
Academic and research departments
Leverhulme Quantum Biology Doctoral Training Centre (QB-DTC), School of Biosciences, Faculty of Health and Medical Sciences, Faculty of Engineering and Physical Sciences.About
My research project
Exploring the role of noise on quantum decoherence in cellular systemsNoise, defined as the standard deviation normalised to the mean of any set of observations, is a fundamental challenge in quantitative models of molecular biology. Michael will focus on characterising noise in a model organism and connecting observed noise to a novel cause of noise, post-transcriptional regulation. The 3’ untranslated region (UTR) of mRNA transcripts has been highlighted in previous work as a possible region for translational auto-regulation by corresponding RNA-binding proteins[4]. He will generate next-generation mNeonGreen-tagged strains in the model organism Saccharomyces cerevisiae. The strains will improve on previous strains by preserving the endogenous 3’ UTRs while allowing sensitive quantification of autoregulatory RNA-binding proteins. Quantification will be done using high-throughput flow cytometry, to provide powerful and reliable results. This basic research will give insights into the central dogma of biology and constitutes a missing link in modern models of gene expression for biomedical research and synthetic biosyntheses which focus on transcriptional noise but not yet translation.
Supervisors
Noise, defined as the standard deviation normalised to the mean of any set of observations, is a fundamental challenge in quantitative models of molecular biology. Michael will focus on characterising noise in a model organism and connecting observed noise to a novel cause of noise, post-transcriptional regulation. The 3’ untranslated region (UTR) of mRNA transcripts has been highlighted in previous work as a possible region for translational auto-regulation by corresponding RNA-binding proteins[4]. He will generate next-generation mNeonGreen-tagged strains in the model organism Saccharomyces cerevisiae. The strains will improve on previous strains by preserving the endogenous 3’ UTRs while allowing sensitive quantification of autoregulatory RNA-binding proteins. Quantification will be done using high-throughput flow cytometry, to provide powerful and reliable results. This basic research will give insights into the central dogma of biology and constitutes a missing link in modern models of gene expression for biomedical research and synthetic biosyntheses which focus on transcriptional noise but not yet translation.
Publications
Post-transcriptional gene regulation is driven by RNA-binding proteins (RBPs). Recent global approaches suggest widespread autoregulation of RBPs through binding to their own mRNA; however, little is known about the regulatory impact and quantitative models remain elusive. By integration of several independent kinetic parameters and abundance data, we modelled autoregulatory feedback loops for six canonical and non-canonical RBPs from the yeast Saccharomyces cerevisiae, namely Hrb1p, Hek2/Khd1p, Ski2p, Npl3p, Pfk2p, and Map1p. By numerically solving ordinary differential equations, we compared non-feedback models with models that considered the RPBs as post-transcriptional activators/repressors of their own expression. While our results highlight a substantial gap between predicted protein output and experimentally determined protein abundances applying a no-feedback model, addition of positive feedback loops are surprisingly versatile and can improve predictions towards experimentally determined protein levels, whereas negative feedbacks are particularly sensitive to cooperativity. Our data suggests that introduction of feedback loops supported by real data can improve models of post-transcriptional gene expression.