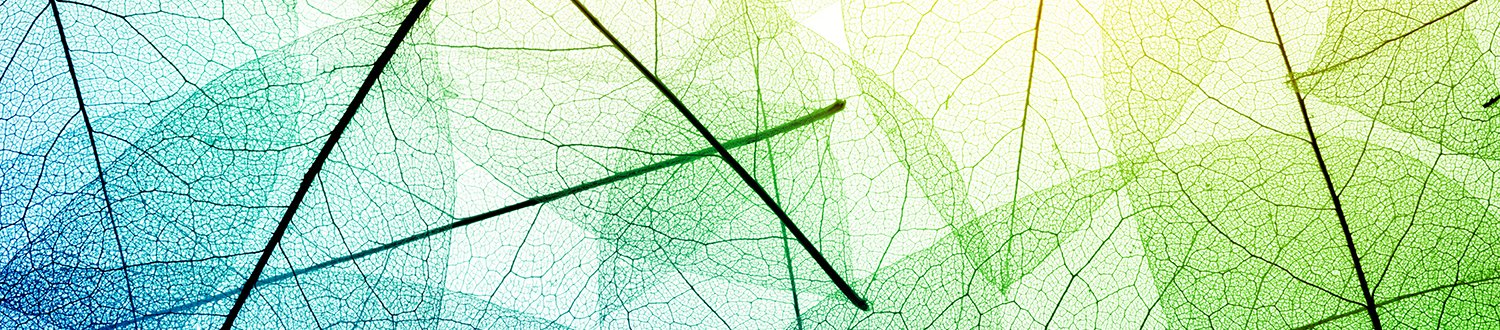
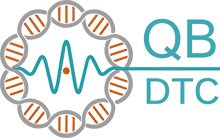
Ion channels
Ion channels are transmembrane proteins specialised in the fine control of the passive flow of naturally occurring ions (Sodium, Chloride, Potassium, and Calcium). They play crucial roles in living cells, from initiating and facilitating propagation of electrical signals across membranes to regulating cell volume. They are so important that they rank as the second most important target for the development of pharmaceutical drugs, yet the basis of ion transportation is still not completely understood, and recent finding shed a light on the involvement of non-trivial quantum effects on the mechanisms underlying this process. Improving our comprehension of ion transportation will be key to ameliorate our understanding of the very essence of cellular homeostasis and communication.
Overview
Properties central to the functionality of many ion channels are ion selectivity and ion conduction. Ion selectivity is conferred by a portion of the protein known as the selectivity filter; these filters provide the ability to discriminate between different ions due to its diameter (of a few angstroms) and highly conserved motifs of residues that pull ions within the pore thanks to the attractive force of Coulomb interactions. This ensures that ions proceed through the channel in a single file fashion without their hydration shell. Ion conduction on the other hand, is regulated by a specialised segment known as the voltage-sensing domain that can modify the opening state of the whole protein in response to a change of voltage in the membrane.
Both selectivity and conduction have been recently proposed to be influenced by quantum coherence and quantum interference. Indeed, many ion channels display remarkable values of conduction and selectivity that cannot be successfully explained by current models, as in the case of bacterial voltage-gated potassium channel KcsA, combining very high throughput rates (108 ions/sec.), close to the diffusion limit, with a high discrimination rate between potassium and sodium (104:1), despite of the low difference between the Potassium and Sodium atomic radius (0.38 Å).
Here at the QB-DTC we will use different techniques and models to test the hypothesis that non-trivial quantum effects are required for a satisfactory comprehension of the mechanisms underlying ion transportation.
Current projects
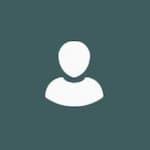
Investigation of quantum coherent transport and EM field sensitivity of ion channel transport of neuronal cells
Federico Bertagna
Postgraduate researcher
Project description
Nerve ion channels play a crucial role in brain function as nerve firing is triggered by the opening and closing of ion channels that allows a current of molecular ions to flow in and out of neurons, triggering an action potential to travel along the nerve. It has been hypothesized that quantum coherence and quantum interference effects play a key role in both selectivity and speed of transport through ion channels in nerve membranes. This hypothesis seems to correlate with the recent finding that weak electromagnetic (EM) fields, of the strength and structure of endogenous EM fields in the brain, influence the pattern of neuron firing and particularly neuronal synchrony. The neuronal synchrony may also be associated with entanglement of EM signals, as well as multi-bit level parallel processing due to the wave nature of the EM signal and the ion channel when triggered.
The aim of this project is to test the hypothesis that quantum coherence plays a role in neuronal ion transport and investigate its sensitivity to EM fields. We will thus start to investigate the effects of different EM fields on different ion channels. We will use electrophysiological techniques, such as Patch Clamp, to record currents through ion channels both with and without external EM fields and using both normal and heavy isotopes of ions, such as Na+, K+ and other relevant ions, in order to perturb coherences. We will also attempt to examine if more than one EM signal coupled to the trigger will allow for the firing to be made easier due to lower thresholds via an entanglement mechanism. We will compare the results to predictions made through quantum mechanical simulations of ion channel conductivity in order to test the hypothesis that quantum coherence is involved in ion channel conductivity and selectivity.
Supervisors
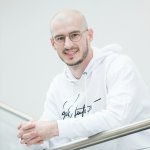
Exploration of ion selectivity in the Epithelial Sodium Channel
Cedric Vallee
Postgraduate researcher
Project description
The aim of this exciting PhD is to investigate selectivity of sodium (Na+) within a specific channel: the Epithelial Sodium Channel (ENaC). Studies will be driven by two different but related approaches: simulation via computer modelling and experiments via electrophysiology. I will mainly be focused on the selectivity filter of the channel, which is the part where the channel can discriminate different ions, even those of identical charge, allowing only the right one to pass through, in this case Na+. The motif in the ENaC is (G/S)XS, where X is a different residue in each subunit and also in other channel of the same family like ASIC for example.
It has been stated that residues of the selectivity filter create an energy barrier that ions have to cross. The aim of my project is to simulate the ion flow through these energy barriers, whilst experimentally measuring ENaC kinetics. To investigate the mechanism, several parameters can be tested: different ions (Li+, K+, Ca2+), isotopes (24Na, 40K, heavy water), flow direction and phospholipid composition of the membrane.
Supervisors
Research team
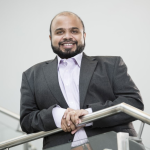
Professor Kamalan Jeevaratnam
Head of School of Veterinary Medicine, Professor in Clinical Physiology
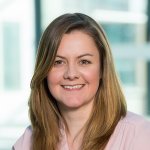
Dr Rebecca Lewis
Senior Lecturer in Physiology
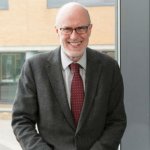
Professor Johnjoe McFadden
Professor of Molecular Genetics, Associate Dean (International)
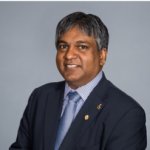
Professor Ravi Silva
Director, Advanced Technology Institute (ATI) and Head of NanoElectronics Centre