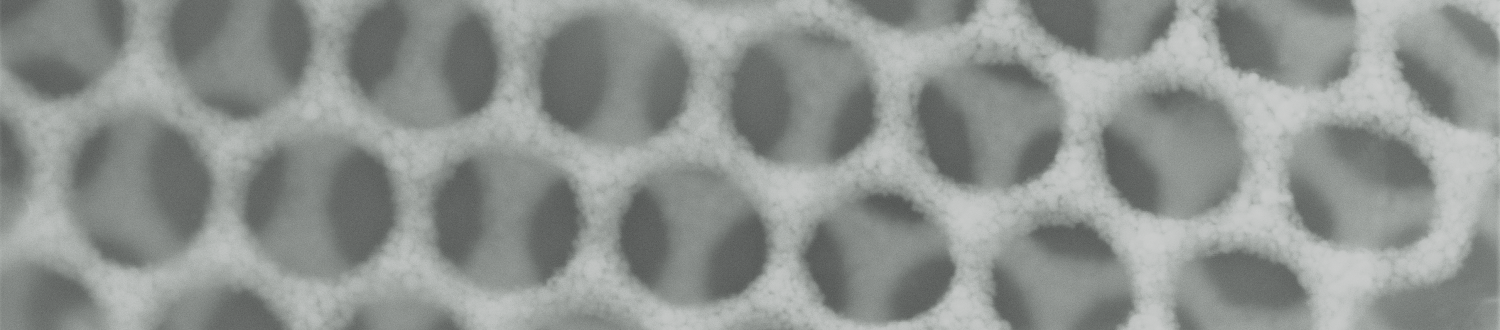
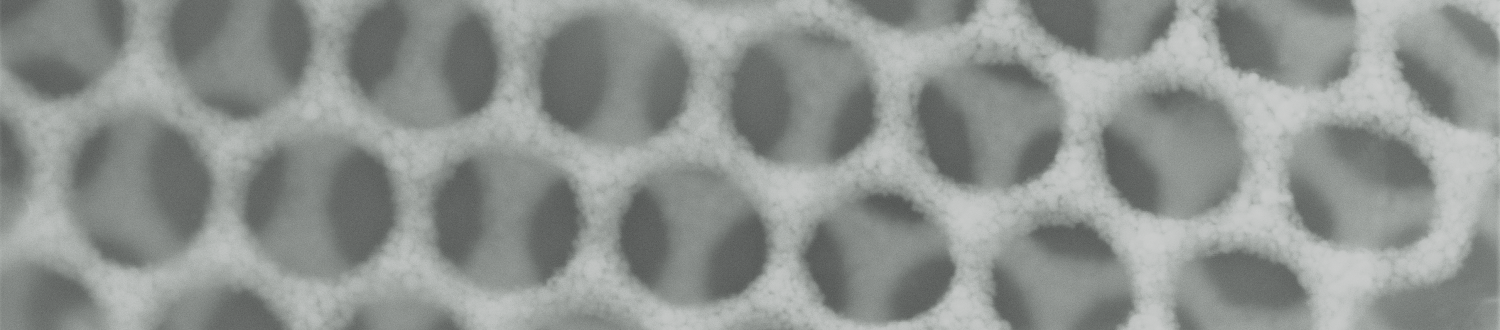
Projects
Below are PhD projects offered by group members. If you interested in one, please drop the supervising academic an email.
Possible PhD projects
Our group is highly international, we have present and past PhD students and postdocs from Italy, Spain, Malaysia, India, Saudi Arabia, Greece, France, Germany, Poland, Hungary, Malta, Thailand and China, as well as the UK.
- Supervisor - Richard Sear, Lindsay Broadbent, Al Haddrell (Bristol), Wooli Bae
- Type - experimental/interdisciplinary
Project description
Pandemics are caused by respiratory viruses (COVID-19, flu) that spread in aerosols. These pandemics hit us at a rate of about one pandemic per century, and cause about 10 million deaths/pandemic. Despite this, we know surprisingly little about how viruses spread in aerosols. The PhD will use state-of-the-art experimental aerosol science and molecular biology to investigate the mechanism by which viruses are destroyed by the sudden drying in an aerosol. For example, is it the virus’s spike protein that is destroyed by drying? The project is interdisciplinary in nature. It will involve producing proteins and viruses, and quantifying their survival after aerosol drying.
This project is funded by the EPSRC Centre for Doctoral Training in Aerosol Science. So please go here for the application procedure.