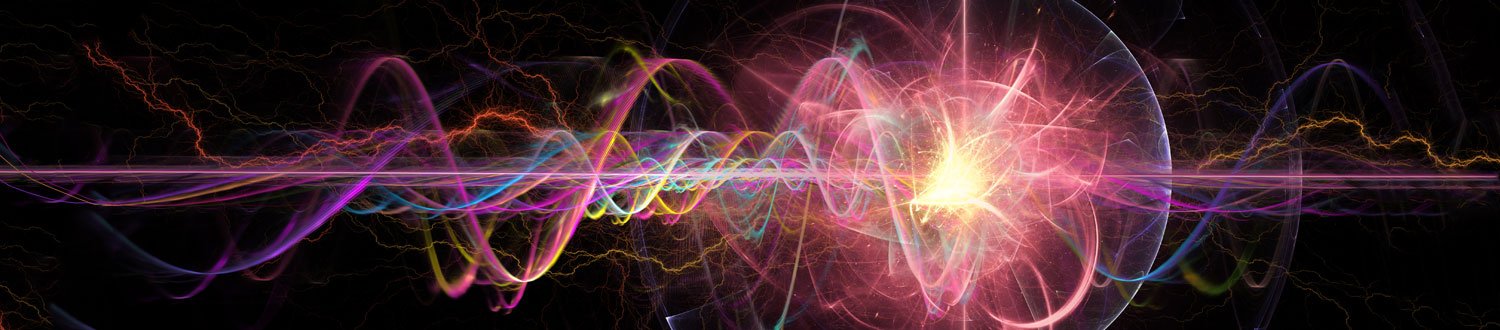
Quantum foundations
Our group is interested in tackling various problems in the foundations of quantum mechanics, from the fundamental role of quantum entanglement in the emergence of an arrow of time and the different ways of measuring quantum entropy to the connection between the quantum and classical worlds.
Research leads
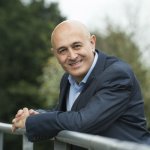
Professor Jim Al-Khalili
Professor
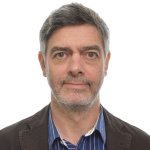
Professor Dorje Brody
Professor
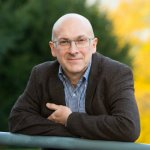
Dr Andrea Rocco
Associate Professor
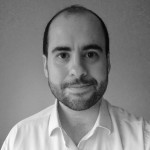
Dr Jesús Rubio Jiménez
Surrey Future Fellow
Overview
Our research into the origin of time irreversibility in open quantum systems has provided fertile ground for new theoretical advances in quantum thermodynamics and the notion of quantum entropy. We are interested in addressing the emergence of the arrow of time using a range of probabilistic approaches, such as fluctuation theorems and logical inference.
Since the early days in the development of quantum mechanics, the interaction of classical and quantum systems emerged as a fundamental open question mainly motivated by Bohr's description of quantum measurement. Over the decades, the problem of classical-quantum coupling has made its appearance in a variety of contexts, ranging from measurement theory to quantum gravity and from chemical physics to quantum biology.
Much of this work is funded by the John Templeton Foundation Project Life on the Edge: quantum thermodynamics, quantum biology and the Arrow of Time.
Research projects
Quantum decoherence arrow of time

In quantum mechanics, we appeal to decoherence as a process to explain the emergence of a quasi-classical order. Decoherence, whose origin lies in quantum entanglement, has no classical counterpart. Moreover, it is an irreversible process.
This is a theoretical project in the foundations of physics that will explore the origin of irreversibility for an open quantum system (that is, time pointing in the direction of increasing entanglement) and whether it is similar to the origin of irreversibility in thermodynamics, namely that there is some measure of entanglement entropy for the universe that started off in a special initial state in the same way that Boltzmann’s thermodynamic entropy requires a ‘past hypothesis’. And since the quantum state of the universe is the only truly closed system (evolving according to the unitary dynamics of the Schrödinger equation), can we define an entanglement arrow of time for it even though it has no external environment to entangle with?
The problem of how the irreversibility of time emerges from time- reversal invariant dynamical equations, whether classical or quantum (often referred to as Loschmidt’s paradox) has been around for so long that many physicists and philosophers either assume it has been resolved or that it is too intractable and therefore uninteresting.
Of late it has come back into fashion, motivated by interest in fields such as quantum information theory, non-equilibrium statistical mechanics and quantum thermodynamics, as well as ongoing puzzles such as the black hole information paradox, entropic gravity and the search for a theory of quantum gravity.
Quantum measurements for fundamental physics
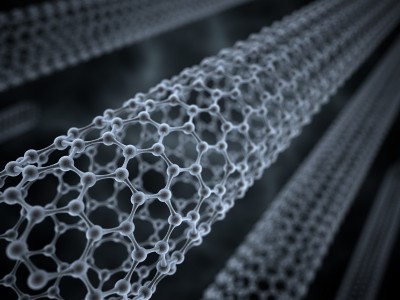
Progress in the foundations of physics demands a theory of measurements capable of accessing regimes where fundamental physics comes into play. From testing the equivalence principle, or exploring the interplay between Lorentz invariance and quantum entanglement, to devising effective ways of manipulating information in the nanoscale, or in space, modern quantum metrology offers the best framework to model and explain measurements in physics.
One of our research lines is devoted to this task.
Quantum theory of the cognitive state of mind
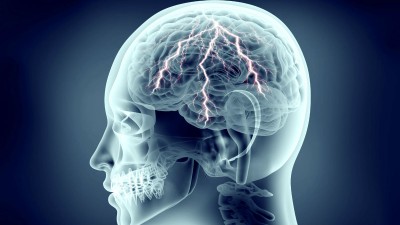
One of the most influential debates in cognitive science concerns the clash between probabilistic prescription and human behaviour. It seems reasonable that behaviour follows rules of classical probability, but there are also surprising apparent fallacies in behaviour, such as the conjunction fallacy, whereby naive observers confidently decide that a conjunction is more likely than a marginal.
Behavioural science has attracted three Nobel prizes, all relating to the friction between normative expectations for behaviour based on classical probability theory and apparent probabilistic fallacies. The failure of human behaviour to comply with classical probability principles is reminiscent of the failure observed a century ago of physical systems to comply with the laws of classical physics. Astonishingly, in both cases, the rules of quantum theory can adequately describe classically problematic empirical data.
This observation has led to the development of a new research field called ‘quantum cognition’ within cognitive science. Much of the literature on quantum cognition has focused on the effects resulting from incompatible statements, as represented by non-commutative variables of quantum theory.
Relatively little attention has been paid to the role of dynamics or quantum entanglement in affecting cognitive behaviours, and these are the topics we examine and explore. Ultimately, this can lead to the notion of an artificial quantum intelligence whereby the AI architecture follows quantum rules.
Computational efficiencies of green plants
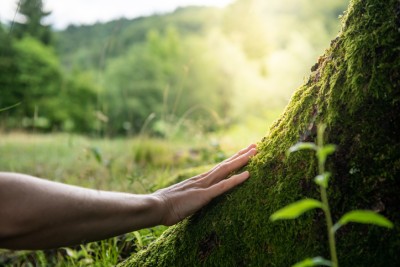
Mankind is facing the greatest ever challenge to its prosperity from the rapid and devastating advancement of the impacts of climate change. The threats caused by climate change are multitudinous, predominant among them is food security. Green plants form the basis of almost all food chains on earth, but conditions for their growth are changing too rapidly for them to adapt.
Understanding efficiencies and capabilities of plants is acutely important, and this demands the development of a novel mathematical framework that enables us to infer conditions under which plants can adapt to changing environmental conditions. By applying and extending the mathematical theory of quantum signal detection, one can characterise dynamic behaviours of green plants. These models in turn enable us to measure information contents of environmental messages experienced by plants, and to estimate the computational efficiencies of plants.
Such estimates are important because different species are likely to process information with different efficiencies, with considerable consequences for agriculture; for an ecological understanding of invasive plants; and more generally for responses to environmental changes that lead to ecological stability.
Now if there are conflicting environmental cues reaching a green plant then the classical theory of signal detection fails to capture the dynamical features adequately. Nevertheless, their quantum counterpart represented by certain class of stochastic Schrödinger equations, are able to model such situations.
This project therefore explores computational capabilities of green plants (or other biological systems), while at the same time examines mathematical structures and physical implications of stochastic Schrödinger equations that give rise to Lindblad equations of open quantum systems.