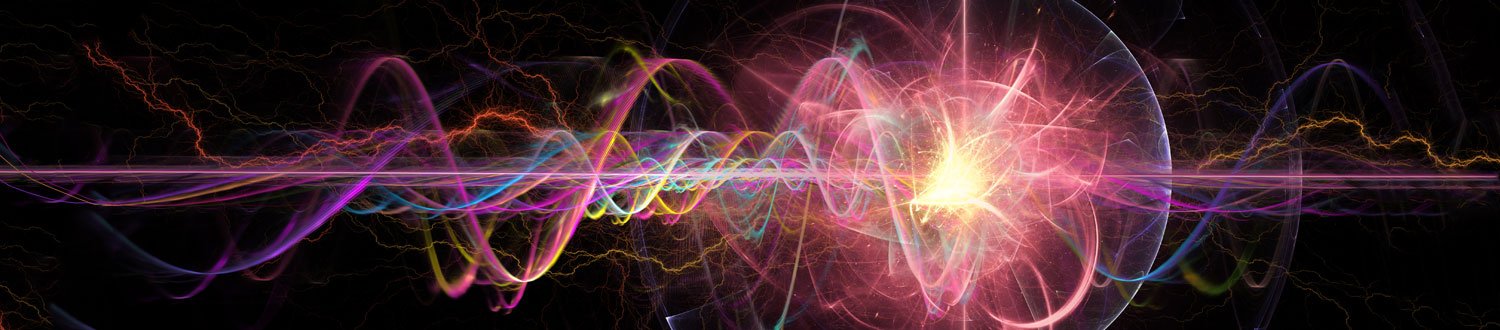
Quantum chemistry and quantum biology
The University of Surrey is now established as a world leading centre in the new interdisciplinary field of quantum biology. Members of our group collaborate with colleagues in chemistry and molecular biology to investigate the potential role of quantum mechanics in living systems.
Research leads
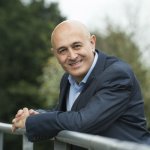
Professor Jim Al-Khalili
Professor
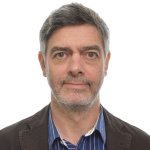
Professor Dorje Brody
Professor
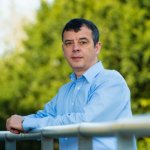
Professor Marian Florescu
Professor
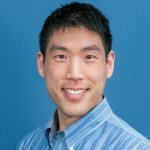
Dr Youngchan Kim
Lecturer
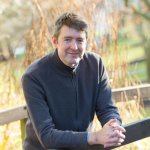
Professor Benedict Murdin
Professor
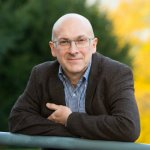
Dr Andrea Rocco
Associate Professor
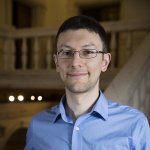
Dr Marco Sacchi
Associate Professor
Overview
One of the biggest unresolved mysteries in science is the way living organisms maintain their highly ordered, low entropy, far-from-equilibrium state inside living cells. Already, a number of examples in biology have been discovered that seem to need a helping quantum hand, making use of no-trivial quantum mechanisms, such as superposition, tunnelling and entanglement. To understand how life is able to maintain the delicate quantum coherences that allow for such behaviour at the molecular scale to have a functional role, we need to model them as open quantum systems strongly coupled to their surrounding environments.
In his 1944 book What is Life?, Erwin Schrödinger argued that organisms stay alive precisely by staving off equilibrium. It does this by taking in low entropy free energy from its environment. In this way, life is able to maintain structure and complexity. However, this ability must be traced down to the molecular level and, ultimately, the quantum domain. So, is there something even more profound going on inside living systems than the mere exploitation of the rules of quantum mechanics?
Much of this work is funded by the John Templeton Foundation Project Life on the Edge: quantum thermodynamics, quantum biology and the Arrow of Time and the Leverhulme Quantum Biology Doctoral Training Centre.
Computational chemistry
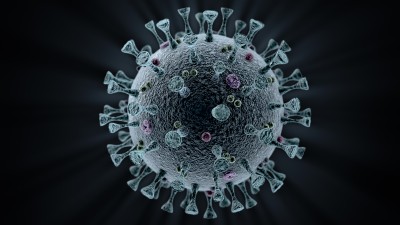
The role of computational biochemistry in understanding and quantifying the quantum nature of life and biological systems cannot be overestimated. At Surrey, in the last five years, we have paved the way for innovative approaches in this area, in particular for understanding the role of proton tunnelling and de-localisation in the creation of point defects in DNA, which could have implications for cancer research as well as helping us understand the rate of evolution.
There is still considerable work that we can do using computational approaches, which include quantum chemistry combined with multi-scale simulations and open quantum systems. Among those “long-term goals” there are:
- The definition and calculation of entropy in biological systems, especially in DNA, with its connection with information theory and quantum entropy.
- The role of quantum effects in propagating or correcting genetic errors.
- The interaction of light and electromagnetic radiations with DNA and their role in generating DNA damage through coherent quantum processes.
In order to progress in the above-mentioned long-standing problems, computational methods need to be applied in conjunction with state-of-the-art experimental techniques, in particular time-resolved spectroscopy and ultrafast scattering techniques.
Close collaboration with mathematical and theoretical physics research is also essential as new methods and algorithms need to be developed to elucidate the connection between purely quantum particles such as electrons and protons with “classical” environments such as proteins, solvents and ligands.
Proton tunnelling in DNA in an open quantum system approach
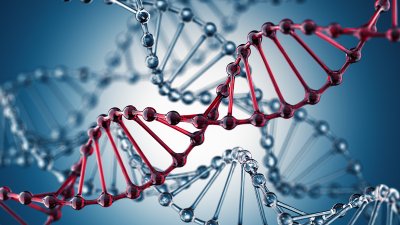
One of the most vibrant areas of research in quantum biology, and in which the team at Surrey is world leading, is the study of proton tunnelling in DNA. Proton transfer across hydrogen bonds in DNA can produce non-canonical nucleobase dimers and is a possible source of single-point mutations when these forms mismatch under replication.
Previous computational studies by the group have revealed this process to be energetically feasible for the guanine-cytosine (GC) base pair, but the tautomeric product (GC) is short-lived.
Using a combination of computational chemistry (density functional theory and multi-scale quantum mechanical/molecular dynamics (QM/MM) simulations) together with an open quantum systems approach to account for the cellular environment, we model this process, including the effect of the replisome enzymes on proton transfer during strand separation and replication.
Dynamical decoherence and memory effects in green fluorescent proteins
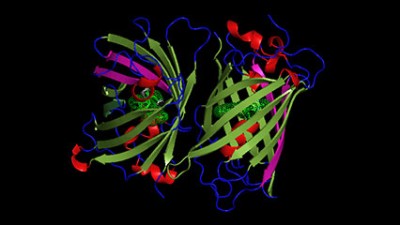
The interaction of a quantum system with its surroundings has major implications on its evolution and can significantly limit our ability to exploit quantum degrees of freedom for advanced applications. Understanding the environment-mediated tendency of quantum mechanical systems to decohere and lose their non-classical correlations is of utmost importance and may open avenues yet to be explored for addressing basic physics questions and practical quantum technology applications.
In parallel, in the emerging field of quantum biology, there is a growing body of evidence that in certain bio-molecular complexes, quantum effects dominate over their purely classical correspondents, especially in the case of photo-excited systems for which the surrounding dielectric environment plays an essential role in the system dynamics.
We are currently exploring the physical phenomena associated with the dynamical decoherence of the chromophores within a green fluorescent protein coupled to a finite-temperature dielectric environment, a system of significant interest due to its anomalously long coherence lifetimes.
We are focusing our investigation on the degree of coherence displayed by independent green fluorescent protein chromophores and the energy transfer dynamics mediated by the dielectric relaxation of the environment with the aim of understanding long coherence lifetimes in these systems.