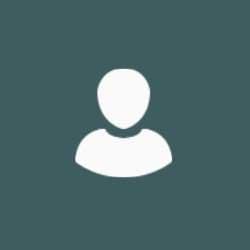
Dr Jing Li
About
My research project
Synthesis and Microstructural Analysis of Modified Apatite type Lanthanum silicate (LSO) electrolyte for LT SOECsUsing co-precipitation method to synthesise the apatite type LSO as the electrolyte for LT-SOEC for hydrogen production. And using co-doping and composition methods to improve the conductivity.
Supervisors
Using co-precipitation method to synthesise the apatite type LSO as the electrolyte for LT-SOEC for hydrogen production. And using co-doping and composition methods to improve the conductivity.
Publications
This is an extensive review on the application of thermally sprayed coatings with functional properties for electrolysers. Such coatings are critical and vital constituents (as catalysts (anode/cathode), solid electrolyte, and transport layer, including corrosion-prone parts such as bipolar plates) of the water splitting electrolysis process for hydrogen production. Thermal spray coatings have the advantage of providing thick and functional coatings from a range of engineering materials. The associated coating processes provide good control of coating thickness, morphology, microstructure, pore size and porosity, and residual strain in the coatings through selection of suitable process parameters for any coating material of interest. This review consolidates scarce literature on thermally sprayed components which are critical and vital constituents (e. g., catalysts (anode/cathode), solid electrolyte, and transport layer, including corrosion-prone parts such as bipolar plates) of the water splitting electrolysis process for hydrogen production. The research shows that there is a gap in thermally sprayed feedstock material selection strategy as well as in addressing modelling needs that can be crucial to advancing applications exploiting their catalytic and corrosion-resistant properties to split water for hydrogen production. Due to readily scalable production enabled by thermal spray techniques, this manufacturing route bears potential to dominate the sustainable electrolyser technologies in the future. While the well-established thermal spray coating variants may have certain limitations in the manner they are currently practiced, deployment of both conventional and novel thermal spray approaches (suspension, solution, hybrid) is clearly promising for targeted development of electrolysers.
A comprehensive discussion of the approaches for developing carbon-based sulfur hosts is presented, encompassing structural design and functional optimization. The recent implementation of effective machine learning methods in discovering carbon-based sulfur hosts has been systematically examined. The challenges and future directions of carbon-based sulfur hosts for practically application have been comprehensively discussed. A summary of the strengths and weaknesses, along with the outlook on carbon-based sulfur hosts for practical application has been incorporated. As the need for high-energy–density batteries continues to grow, lithium-sulfur (Li–S) batteries have become a highly promising next-generation energy solution due to their low cost and exceptional energy density compared to commercially available Li-ion batteries. Research into carbon-based sulfur hosts for Li–S batteries has been ongoing for over two decades, leading to a significant number of publications and patents. However, the commercialization of Li–S batteries has yet to be realized. This can be attributed, in part, to the instability of the Li metal anode. However, even when considering just the cathode side, there is still no consensus on whether carbon-based hosts will prove to be the best sulfur hosts for the industrialization of Li–S batteries. Recently, there has been controversy surrounding the use of carbon-based materials as the ideal sulfur hosts for practical applications of Li–S batteries under high sulfur loading and lean electrolyte conditions. To address this question, it is important to review the results of research into carbon-based hosts, assess their strengths and weaknesses, and provide a clear perspective. This review systematically evaluates the merits and mechanisms of various strategies for developing carbon-based host materials for high sulfur loading and lean electrolyte conditions. The review covers structural design and functional optimization strategies in detail, providing a comprehensive understanding of the development of sulfur hosts. The review also describes the use of efficient machine learning methods for investigating Li–S batteries. Finally, the outlook section lists and discusses current trends, challenges, and uncertainties surrounding carbon-based hosts, and concludes by presenting our standpoint and perspective on the subject.
Extreme fast charging of Ampere-hour (Ah)-scale electrochemical energy storage devices targeting charging times of less than 10 minutes are desired to increase widespread adoption. However, this metric is difficult to achieve in conventional Li-ion batteries due to their inherent reaction mechanism and safety hazards at high current densities. In this work, we report 1 Ah soft-package potassium-ion hybrid supercapacitors (PIHCs), which combine the merits of high-energy density of battery-type negative electrodes and high-power density of capacitor-type positive electrodes. The PIHC consists of a defect-rich, high specific surface area N-doped carbon nanotube-based positive electrode, MnO quantum dots inlaid spacing-expanded carbon nanotube-based negative electrode, carbonate-based non-aqueous electrolyte, and a binder- and current collector-free cell design. Through the optimization of the cell configuration, electrodes, and electrolyte, the full cells (1 Ah) exhibit a cell voltage up to 4.8 V, high full-cell level specific energy of 140 Wh kg−1 (based on the whole mass of device) with a full charge of 6 minutes. An 88% capacity retention after 200 cycles at 10 C (10 A) and a voltage retention of 99% at 25 ± 1 °C are also demonstrated.
Apatite-type lanthanum silicate (LSO) electrolyte is one of the most promising candidates for developing intermediate-temperature solid oxide electrolysis cells and solid oxide full cells (IT-SOECs and SOFCs) due to its stability and low activation energy. However, the LSO electrolyte still suffers from unsatisfied ionic conductivity and low relative density. Here in this work, a novel co-doped method is reported to prepare highly purified polycrystalline powders of Mg-Mo co-doped LSO (Mg/Mo-LSO) electrolytes with high excellent densification properties and improved ionic conductivity. Introducing the Mo 6+ and Mg 2+ ions into the LSO structure can increase the number of interstitial oxide ions and improve the degree of densification at lower sintering temperatures, more importantly, expand the migration channel of oxide ions to enhance the ionic conductivity. As a result, the relative density of the fabricated Mo/Mg-LSO electrolytes pellets could achieve more than 98% of the theoretical density after sintering at 1500 ℃ for 4 h with a grain size of about 1-3 μm and the EIS results showed the ionic conductivity increased from 0.782 mS·cm-1 for the pristine LSO to 33.94 mS·cm-1 for the doped sample La 9.5 Si 5.45 Mg 0.3 Mo 0.25 O 26+δ at 800 ℃. In addition, the effect of different Mo 6+ doping contents was investigated systematically, in which La 9.5 Si 5.45 Mg 0.3 Mo 0.25 O 26+δ possessed the highest ionic conductivity and relative density. The proposed Mo/Mg co-doped method in this work is one step forward in developing apatite-structured electrolytes offering excellent potential to address the common issues associated with the fabrication of dense, highly conductive, and thermochemically stable electrolytes for solid oxide electrolysers and fuel cells.