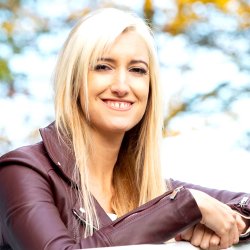
Dr Eva Bestelink
About
Biography
Dr Eva Bestelink is a postdoctoral researcher working with Associate Professor Dr Radu Sporea on contact-controlled thin-film transistors and circuits for large area electronics. Her research is mainly focused on source-gated transistors and the multimodal transistor, which she co-invented with Dr Sporea during her second year as an undergraduate BEng EEE student at the University of Surrey.
During her PhD, she won the 2021 IET Postgraduate Prize for Execllence and Innovation in Engineering.
My qualifications
Affiliations and memberships
News
ResearchResearch interests
Eva's research involves exploring contact-controlled transistors and phototransistors in a variety of thin-film technologies, on rigid and flexible substrates. She helps lead several international collaborations, as well as patent commercilaization efforts.
Research interests
Eva's research involves exploring contact-controlled transistors and phototransistors in a variety of thin-film technologies, on rigid and flexible substrates. She helps lead several international collaborations, as well as patent commercilaization efforts.
Teaching
During her PhD at University of Surrey, Eva contributed to teaching activities within the EEE department, assisting with 1st year UG laboratory demonstration for EEE1027 LDPS I and EEE1028 LDPS II modules. She also assisted with supervision of EEE 3017 Year 3 Projects, particularly inlolving device simulation and/or fabrication.In addition, Eva co-supervised SATRO summer placement students for simulation based projects.
Eva and her PhD supervisor, Dr Radu Sporea, jointly presented "Beyond learning outcomes: Synergy through frank experience-based communication,” at ExciTES April, 2019 - Excellence in Teaching Symposium, as well as "Stag Audio - The Online Electronics Lab” at ExciTES 2021, led by Dr George Bairaktaris, during his PhD.
Publications
We report the first implementation of a complementary circuit using thin-film source-gated transistors (SGTs). The n-channel and p-channel SGTs were fabricated using the inorganic and organic semiconductors amorphous InGaZnO (IGZO) and dinaphtho[2,3- b :2′,3′- f ]thieno[3,2- b ]thiophene (DNTT), respectively. The SGTs exhibit flat output characteristics and early saturation (d V DSAT /d V GS = 0.2 and 0.3, respectively), even in the absence of lateral field-relief structures, thanks to the rectifying source contacts realized with Pt and Ni, respectively. Hence, the complementary inverter shows excellent small-signal gain of 368 V V −1 and noise margin exceeding 94% of the theoretical maximum. We show that the trip point of such inverters can be tuned optically, with interesting applications in compact detectors and sensors. Numerical simulation, using Silvaco ATLAS, reveals that optimized and monolithically-integrated SGT-based complementary inverters may reach a small-signal gain over 9000 V V −1 , making them highly suited to low and moderate speed digital thin-film applications. This proof-of-concept demonstration provides encouraging results for further integration and circuit level optimizations.
Abstract With growing interest in organic phototransistors, as not only sensors but also neuromorphic computing elements, the vast majority of research investigates structures comprising Ohmic source/drain contacts. Here, it is shown how source‐gated transistors (SGTs), in which a source contact barrier dominates electrical characteristics, can be implemented as phototransistors. Organic photo‐SGTs (OPSGTs) based on vacuum‐processed small‐molecule dinaphtho[2,3‐b:2′,3′‐f]thieno[3,2‐]thiophene (DNTT) demonstrate low saturation voltage, exceptional tolerance to channel length variation, and photo‐to‐dark current ratio (PDCR) peaks over 10 6 for 819 µW broad spectrum incident light power. At zero gate‐source voltage, the PDCR reaches 10 4 , showing promise for simple sensor circuit implementation in medical and wellbeing applications.
We have recently demonstrated that, contrary to conventional TFT design rules, emissive pixels based on source‐gated transistor drivers benefit from choosing a source‐gate overlap of several microns. Using TCAD simulations we show that incorporating the SGT overlap capacitance with that of the conventional storage capacitor, pixel area can be optimized, with no adverse impact on data retention. For the technology considered, the optimum source‐gate overlap was 4 ‐ 8 μm, which is in the range of highest operating frequencies for source‐gated transistors.
A Corial Inductively Coupled Plasma Chemical Vapor Deposition (ICP‐CVD) system has been investigated to produce un‐doped and doped μ‐Si layers, as well as insulators, leading to a general capability of performing N and P type TFTs. This enables to develop rapid prototyping of TFTs. Resistivity of layers and TFT issues from ICP‐CVD have been electrically characterized.
Silicon-based digital electronics have evolved over decades through an aggressive scaling process following Moore’s law with increasingly complex device structures. Simultaneously, large-area electronics have continued to rely on the same field-effect transistor structure with minimal evolution. This limitation has resulted in less than ideal circuit designs, with increased complexity to account for shortcomings in material properties and process control. At present, this situation is holding back the development of novel systems required for printed and flexible electronic applications beyond the Internet of Things. In this work we demonstrate the opportunity offered by the source-gated transistor’s unique properties for low-cost, highly functional large-area applications in two extremely compact circuit blocks. Polysilicon common-source amplifiers show 49 dB gain, the highest reported for a twotransistor unipolar circuit. Current mirrors fabricated in polysilicon and InGaZnO have, in addition to excellent current copying performance, the ability to control the temperature dependence (degrees of positive, neutral or negative) of output current solely by choice of relative transistor geometry, giving further flexibility to the design engineer. Application examples are proposed, including local amplification of sensor output for improved signal integrity, as well as temperature-regulated delay stages and timing circuits for homeostatic operation in future wearables. Numerous applications will benefit from these highly competitive compact circuit designs with robust performance, improved energy efficiency and tolerance to geometrical variations: sensor front-ends, temperature sensors, pixel drivers, bias analog blocks and high-gain amplifiers.
A new device, the Multimodal Transistor (MMT), separates charge injection from conduction. With design optimization, it can achieve a constant transconductance with independent on/off switching of output current. This functionality has ample applications in energy efficient analog computation and hardware learning.
This study aims to increase the output current of the a-IGZO source-gated transistor (SGT) through TCAD simulation and experiment. As SGT proves to be useful in various low-power applications and wearable devices, the low output current characteristics limit the adaptability of this structure. It is estimated that a higher level output current is achievable by optimizing this architecture while retaining fast saturation characteristics. The SGT structure simulations have been performed with adjusted density- of-states (DoS) parameters from experiments; this made TCAD simulation more realistic and can be used to predict the results of fabricated SGTs. The results from this study show that longer source-channel overlap and thinner channel is preferable; the SGT with longer source-channel overlap will result in the Mode II injection dominating over the Mode I injection. The Mode I injection occurs at the edge of source contact near the channel region, while the Mode II injection occurs at the bulk of the source at the farthest region from the channel. The result from experiment shows that the fabricated SGT exhibits Mode II injection characteristics-that is the current will increase linearly with increasing gate voltage. From this study. it could be concluded that the source-channel overlap of 210 µm with a channel thickness of 20 nm and channel length of 5 µm is the optimized structure that could provide high output current with little temperature dependence. Further improvement of the output current could be achieved by utilizing lower work function source metal.
Multimodal transistors (MMTs) build on previous understanding of contact‐controlled transistors. Additionally, they allow precise control of the charge injected, distinct from controlling the state of conduction of the semiconductor channel. This approach leads to interesting functionality, of benefit to displays and other large area electronic circuits. Here, we summarize the construction and functionality of the MMT and outline potential applications.
A new device, the Multimodal Transistor (MMT), separates charge injection from conduction and achieves a linear dependence of drain current on its control gate voltage. This functionality is used to implement a highly compact digital‐to‐analog‐converter, capable of performing 3‐level, 3‐bit conversion with minimal error (1.2% of LSB).
Thin-film transistors deliberately comprising rectifying source contacts have attractive properties for sensor and driver circuits: high performance uniformity and geometrical tolerance; superior saturation; and high intrinsic gain. The paper reviews the source-gated and multimodal thin-film transistor configurations, and presents their proposed applications to ultra-compact sensing and data processing circuits. Source-gated transistors with nanoscale tunneling contacts offer an alternative to the Schottky-contact fabrication route, which presents processing challenges. Emerging multimodal transistors overcome limitations of traditional contact-controlled devices and add to the list of useful properties: high gain or constant transconductance by design; immunity to drain voltage variations in floating gate configuration; and a significantly faster response time than source-gated transistors. These devices form the foundation for the design of compact, yet extremely versatile, thin-film circuits for sensing, signal conditioning and signal conversion. Finally, a vision is presented in which the properties of these circuits will be essential to convey seamless user interactivity to physical objects, transforming them into intuitive user interfaces beyond traditional displays screens.
Hot-carrier effects are a persistent challenge for Ohmic contact, high carrier mobility thin-film transistors. As semiconductor properties are systematically improved, such phenomena (e.g., the kink effect) are becoming apparent even in materials such as InGaZnO. Few of the past solutions are practical in these low-complexity semiconductor systems. Here, it is shown that contact-controlled devices offer robust performance under extreme biasing conditions due to their distinctive charge injection processes. The recently-developed multimodal transistor (MMT) provides further control still, by separate regulation of current flow and magnitude. Internal electric field distributions in the source and drain regions are studied via technology computer-aided design simulations, and support the formulation of operational guidelines for the MMT's channel control gate for optimal characteristics in saturation. As MMT principles are universal, these findings should inform device design and operation in all high carrier mobility material systems.
Contact-controlled devices, such as source-gated transistors (SGTs), deliberately use energy barriers at the source, and naturally, the positive temperature dependence (PTD) of drain current can be utilized for temperature sensing. We exploit the difference in drain current activation energy, which arises with contact doping in polysilicon n-type contact-controlled transistors, to demonstrate output current with either a PTD or negative temperature dependence (NTD). The range over which output current varies linearly with temperature, as well as the sensitivity, can be tailored by the choice of reference current magnitude and relative source contact properties within the current mirror. The sensing scheme simplifies the circuit design because it relies solely on thin-film transistors and it has inherent immunity to output voltage variation. This ability to tune the sign of temperature dependence allows facile integration in applications requiring homeostasis via feedback, e.g., electronic skin, in a minimal layout area and potentially with convenient reduction of patterning steps during fabrication.
New materials and optimized fabrication techniques have led to steady evolution in large area electronics, yet significant advances come only with new approaches to fundamental device design. The multimodal thin‐film transistor introduced here offers broad functionality resulting from separate control of charge injection and transport, essentially using distinct regions of the active material layer for two complementary device functions, and is material agnostic. The initial implementation uses mature processes to focus on the device's fundamental benefits. A tenfold increase in switching speed, linear input–output dependence, and tolerance to process variations enable low‐distortion amplifiers and signal converters with reduced complexity. Floating gate designs eliminate deleterious drain voltage coupling for superior analog memory or computing. This versatile device introduces major new opportunities for thin‐film technologies, including compact circuits for integrated processing at the edge and energy‐efficient analog computation.
A novel compact realization of the xnor/xor function is demonstrated with multimodal transistors (MMTs). The multimodal thin-film transistors (MMT's) structure allows efficient use of layout area in an implementation optimized for unipolar thin-film transistor (TFT) technologies, which may serve as a multipurpose element for conventional and emerging large-area electronics. Microcrystalline silicon device fabrication is complemented by physical simulations.
Contact-controlled transistors are rapidly gaining popularity. However, simply using a rectifying source contact often leads to unsatisfactory operation, merely as a thin-film transistor with low drain current and reduced effective mobility. This may cause otherwise promising experiments to be abandoned. Here, we analyse data from literature in conjunction with devices we have recently fabricated in polysilicon, organic and oxide semiconductors, highlighting the main factor in achieving good saturation, namely keeping saturation coefficient γ well below 0.3. We also discuss secondary causes of suboptimal electrical characteristics. Correct design of these alternative device structures will expedite their adoption for high gain, low-frequency applications in emerging sensor circuits.
Artificial neural networks (ANNs) providing sophisticated, power-efficient classification are finding their way into thin-film electronics. Thin-film technologies require robust, layout-efficient devices with facile manufacturability. Here, we show how the multimodal transistor's (MMT's) transfer characteristic, with linear dependence in saturation, replicates the rectified linear unit (ReLU) activation function of convolutional ANNs (CNNs). Using MATLAB, we evaluate CNN performance using systematically distorted ReLU functions, then substitute measured and simulated MMT transfer characteristics as proxies for ReLU. High classification accuracy is maintained, despite large variations in geometrical and electrical parameters, as CNNs use the same activation functions for training and classification.
Two-transistor zero-VGS amplifiers made with polysilicon source-gated transistors achieve voltage gain approaching 300 (49dB). TCAD simulations reveal the effect of load and driver transistor geometry on gain and operating frequency. The SGT circuits have simultaneously superior gain and reduced layout area (two-transistor, channel length L = 3μm and width W = 10 and 30μm), relative to conventional TFT implementations. These results recommend low-complexity, compact SGT designs for flexible and printed amplifiers, such as bio- and chemical sensors.
Despite rapidly expanding interest in thin-film source-gated transistors (SGTs), the high temperature dependence of drain current (TDDC) in devices comprising Schottky source barriers is delaying wide adoption. To reduce this effect, alternative source designs have been theorized. Specifically, introducing additional nanoscale layers at the source contact should facilitate tunneling of charge carriers at the Fermi energy level with negligible TDDC. Here, we fabricate amorphous In₂Ga₂ZnO₇ tunnel-contact SGTs (TCSGTs) with three times lower TDDC than polysilicon transistors with Schottky contacts. Numerical simulations help elucidate the control mechanisms. We show that the potential profile across the semiconductor in the bulk of the source-gate overlap region determines injection current, and the introduction of a thin interfacial layer at the contact reduces the role of the contact metal work function in current control and associated temperature effects. This device architecture adds improved thermal stability to the long list of SGT benefits, including low voltage saturation, power-efficient operation, high intrinsic gain, device-to-device uniformity, and robustness to mechanical and electrical stress.
Recently, hybrid organic-inorganic perovskites have emerged as promising photo-sensing materials for next-generation solution-processed phototransistors, achieving high responsivity, detectivity, and fast response. Here, a phototransistor that can detect visible light using a low-cost, solution processed methylammonium lead iodide/zinc oxide (CH3NH3PbI3/ZnO) heterostructure is reported. While typical ZnO thin-film transistors (TFTs) do not show any photocurrent under visible light illumination, CH3NH3PbI3 (MAPbI(3)) coated ZnO TFTs exhibit substantial photocurrent. Additionally, the optical, morphological, and structural characteristics of the light-absorbing layers are further controlled by altering the precursor ratio of methylammonium iodide and lead (II) iodide (MAI:PbI2), which in turn affects the photosensitivity. Stoichiometric composition (MAI:PbI2 = 1:1) of MAPbI(3) demonstrates optimal characteristics with a responsivity of 234 A/W and a high detectivity of 3.74 x 10(13) Jones under white light illumination. The high photo-responsivity and detectivity result from the combination of the suitable optoelectronic properties of the stoichiometric MAPbI(3) film and its smooth interface with the ZnO channel.
Contrary to conventional design principles, currentdriven pixel drivers based on source-gated transistors (SGTs) achieve their optimal drive current and speed with a deliberate 5 -10μm gate-source overlap. Total pixel circuit area need not increase, as the additional device area can be compensated by reducing the pixel storage capacitor. Numerical simulations demonstrate the viability of SGTs for emissive pixel drivers and high gain, low power, robust circuits for emerging sensor arrays.
We describe the physics of the turn-off mechanism in source-gated transistors (SGTs), which is distinct from that of conventional thin-film field-effect transistors and allows significantly lower off currents, particularly in depletion-mode devices. The “n-type” SGT enters its off state when the potential applied across the semiconductor layer is decreased to low positive values or made negative through the applied gate bias, thus impeding charge injection from the source contact. Measurements on polysilicon devices were supported with TCAD simulations using Silvaco Atlas. Alongside the other known benefits of SGTs, including low saturation voltage, tolerance to process variations, and high intrinsic gain, the ability to efficiently block current at high negative gate voltages suggests that these devices would be ideal elements in emerging thin-film power management and rectification circuits.
Many emerging analog and neuromorphic applications would benefit from a fully linear dependence of a transistor's output on its input for reduced distortion and facile design of linear functions. We show how a new TFT structure, the multimodal transistor, can achieve a linearly dependent drain current in saturation (constant transconductance) with direct proportionality over a large range of input voltages.
The first flexible source-gated transistors (SGTs) in microcrystalline silicon have been fabricated and characterized under bending stress. As SGTs are contact controlled devices, the channel does not modulate drain current, however its geometry has implications for operation. We show how reduced channel length in SGTs helps promote negligible threshold voltage shifts when strain is introduced with a radius of r = 2.5 mm.
Low saturation voltages and extremely high intrinsic gain can be achieved in contact-controlled thin-film transistors (TFTs) with staggered device architecture, enabled by the energy barrier introduced at the source contact. The resulting device, the source-gated transistor (SGT), is limited in its usefulness by the high temperature dependence of the drain current induced by the source energy barrier. Here, the interaction between the thermal characteristics of the source contact and the semiconductor to show drastically reduced temperature dependence for SGTs based on organic semiconductors (OSGTs) is exploited. This extraordinarily weak temperature dependence of the drain current is observed regardless of the height of the source energy barrier (27.8% in OSGTs with Ti contacts compared to 22.1% when using Au contacts, over a 34 K range). The reduction in mobility of the semiconductor offsets an increase in thermionic-field emission of charge carriers at the source. This is a first for SGTs and provides a route to removing one of the last hurdles to their wider adoption. The OSGTs with Ti contacts also demonstrate: drain-current saturation at very low drain-source voltages (saturation factor of 0.22); noteworthy stability after 70 days; and minimal drain-current variation with channel length or illumination.