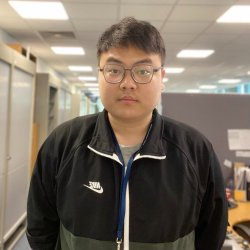
About
My research project
Solid-state batteries and advanced technology for electrochemical characterisationThis project aims to develop new approaches to the design and processing of interfaces in solid-state batteries (SSBs) to underpin critical advances in device performance. A prototype SSB with novel functional artificial interfaces will be developed, featuring low electron/ion interfacial impedance and prevention of dendrite growth and battery over-(dis)charging. Emerging multifunctional characterisation methodologies and modelling will be established by combining focused ion beam scanning electron microscopy (FIB-SEM), tensile stage testing and cutting-edge electrochemical measurements and simulations.
This project aims to develop new approaches to the design and processing of interfaces in solid-state batteries (SSBs) to underpin critical advances in device performance. A prototype SSB with novel functional artificial interfaces will be developed, featuring low electron/ion interfacial impedance and prevention of dendrite growth and battery over-(dis)charging. Emerging multifunctional characterisation methodologies and modelling will be established by combining focused ion beam scanning electron microscopy (FIB-SEM), tensile stage testing and cutting-edge electrochemical measurements and simulations.
Publications
A comprehensive discussion of the approaches for developing carbon-based sulfur hosts is presented, encompassing structural design and functional optimization. The recent implementation of effective machine learning methods in discovering carbon-based sulfur hosts has been systematically examined. The challenges and future directions of carbon-based sulfur hosts for practically application have been comprehensively discussed. A summary of the strengths and weaknesses, along with the outlook on carbon-based sulfur hosts for practical application has been incorporated. As the need for high-energy–density batteries continues to grow, lithium-sulfur (Li–S) batteries have become a highly promising next-generation energy solution due to their low cost and exceptional energy density compared to commercially available Li-ion batteries. Research into carbon-based sulfur hosts for Li–S batteries has been ongoing for over two decades, leading to a significant number of publications and patents. However, the commercialization of Li–S batteries has yet to be realized. This can be attributed, in part, to the instability of the Li metal anode. However, even when considering just the cathode side, there is still no consensus on whether carbon-based hosts will prove to be the best sulfur hosts for the industrialization of Li–S batteries. Recently, there has been controversy surrounding the use of carbon-based materials as the ideal sulfur hosts for practical applications of Li–S batteries under high sulfur loading and lean electrolyte conditions. To address this question, it is important to review the results of research into carbon-based hosts, assess their strengths and weaknesses, and provide a clear perspective. This review systematically evaluates the merits and mechanisms of various strategies for developing carbon-based host materials for high sulfur loading and lean electrolyte conditions. The review covers structural design and functional optimization strategies in detail, providing a comprehensive understanding of the development of sulfur hosts. The review also describes the use of efficient machine learning methods for investigating Li–S batteries. Finally, the outlook section lists and discusses current trends, challenges, and uncertainties surrounding carbon-based hosts, and concludes by presenting our standpoint and perspective on the subject.
Acknowledgements: Y.Z. acknowledges support from EPSRC—New Investigator Award 2020 (EP/V002260/1), The Faraday Institute—Battery Study and Seed Research Project (FIRG052), The Royal Society—International Exchanges 2021 Cost Share (NSFC) (IEC\NSFC\211074). Y. G. thanks the China Scholarship Council (CSC, No. 201806130168). H. L. acknowledges the International Postdoctoral Exchange Fellowship Program (Grant No. PC2022020). Funder: Shanghai Jiao Tong University As the need for high-energy-density batteries continues to grow, lithium-sulfur (Li-S) batteries have become a highly promising next-generation energy solution due to their low cost and exceptional energy density compared to commercially available Li-ion batteries. Research into carbon-based sulfur hosts for Li-S batteries has been ongoing for over two decades, leading to a significant number of publications and patents. However, the commercialization of Li-S batteries has yet to be realized. This can be attributed, in part, to the instability of the Li metal anode. However, even when considering just the cathode side, there is still no consensus on whether carbon-based hosts will prove to be the best sulfur hosts for the industrialization of Li-S batteries. Recently, there has been controversy surrounding the use of carbon-based materials as the ideal sulfur hosts for practical applications of Li-S batteries under high sulfur loading and lean electrolyte conditions. To address this question, it is important to review the results of research into carbon-based hosts, assess their strengths and weaknesses, and provide a clear perspective. This review systematically evaluates the merits and mechanisms of various strategies for developing carbon-based host materials for high sulfur loading and lean electrolyte conditions. The review covers structural design and functional optimization strategies in detail, providing a comprehensive understanding of the development of sulfur hosts. The review also describes the use of efficient machine learning methods for investigating Li-S batteries. Finally, the outlook section lists and discusses current trends, challenges, and uncertainties surrounding carbon-based hosts, and concludes by presenting our standpoint and perspective on the subject.