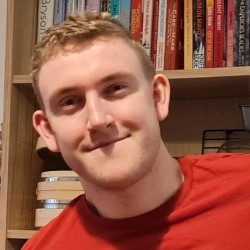
Dr Joseph O'Neill
About
Biography
Dr. Joseph O'Neill received his Ph.D. in 2023, having worked in the Radiation and Medical Physics group for four years specialising in the synthesis, characterisation and device implementation of a range of perovskite scintillators. His doctoral thesis was entitled "Mixed-Cation Perovskite Scintillators for X-Ray Detection".
He joined the Space Environment and Protection in the Surrey Space Centre in August 2023 as a postdoctoral research fellow. His present research interests are Cherenkov-based high-energy proton detectors.
Publications
This article details work performed on the synthesis and characterization of an inorganic mixed-cation double halide perovskite, Cs2Ag.6Na.4In.85Bi.15Cl6 (CANIBIC). Single crystals have been created via a hydrothermal reaction, milled into a powder, and pressed into pellets, while nanocrystals have been directly synthesized via mechanosynthesis. A computational model is constructed to predict the X-ray diffraction pattern of CANIBIC; this model aligns very well with the X-ray diffraction pattern measured for CANIBIC crystal powder. This model can therefore be developed in the future as a tool to predict lattice parameters and crystal structures of other novel double-halide perovskites. Photoluminescence spectra obtained from each format show broad emission centered at 630 nm, as is typical for self-trapped exciton emission; self-trapped exciton emission is also confirmed by investigating photoluminescence intensity as a function of laser power. Nanocomposites are produced via the loading of nanocrystals of CANIBIC into PMMA. Although nanocomposite disks consisting of a small proportion of CANIBIC nanocrystals in PMMA have a smaller mass attenuation coefficient than a pressed pellet of CANIBIC, these disks have comparatively bright radioluminescence due to their optical transparency. These nanocomposite disks are therefore a particularly useful format for the practical use of the CANIBIC scintillator.
We present work on the development of mixed-halide perovskite (CsPbClxBr(1−x)) nanocrystal scintillators for X-ray detection applications. The effect of the varying the halide composition on the resulting peak emission and light yield is discussed, with the CsPbBr3 materials displaying the greatest light yield. These perovskite nanocrystals were successfully loaded into PMMA, an inert plastic, at 2% mass weighting and the responses of these composites were compared to that of their colloidal dispersions. The composites were also characterised in terms of the radioluminescent light yield and decay response, alongside their X-ray sensitivity, in which the PMMA-CsPbBr3 composites again outperformed the materials containing Cl− anions.
We report a facile, solvent-free surfactant-dependent mechanochemical synthesis of highly luminescent CsPbBr3 nanocrystals (NCs) and study their scintillation properties. A small amount of surfactant oleylamine (OAM) plays an important role in the two-step ball milling method to control the size and emission properties of the NCs. The solid-state synthesized perovskite NCs exhibit a high photoluminescence quantum yield (PLQY) of up to 88% with excellent stability. CsPbBr3 NCs capped with different amounts of surfactant were dispersed in toluene and mixed with polymethyl methacrylate (PMMA) polymer and cast into scintillator discs. With increasing concentration of OAM during synthesis, the PL yield of CsPbBr3/PMMA nanocomposite was increased, which is attributed to reduced NC aggregation and PL quenching. We also varied the perovskite loading concentration in the nanocomposite and studied the resulting emission properties. The most intense PL emission was observed from the 2% perovskite-loaded disc, while the 10% loaded disc exhibited the highest radioluminescence (RL) emission from 50 kV X-rays. The strong RL yield may be attributed to the deep penetration of X-rays into the composite, combined with the large interaction cross-section of the X-rays with the high-Z atoms within the NCs. The nanocomposite disc shows an intense RL emission peak centered at 536 nm and a fast RL decay time of 29.4 ns. Further, we have demonstrated the X-ray imaging performance of a 10% CsPbBr3 NC-loaded nanocomposite disc.
Nanocomposite Scintillators
While there is great demand for effective, affordable radiation detectors in various applications, many commonly used scintillators have major drawbacks. Conventional inorganic scintillators have a fixed emission wavelength and require expensive, high-temperature synthesis; plastic scintillators, while fast, inexpensive, and robust, have low atomic numbers, limiting their X-ray stopping power. Formamidinium lead halide perovskite nanocrystals show promise as scintillators due to their high X-ray attenuation coefficient and bright luminescence. Here, we used a room-temperature, solution-growth method to produce mixed-halide FAPbX3 (X = Cl, Br) nanocrystals with emission wavelengths that can be varied between 403 and 531 nm via adjustments to the halide ratio. The substitution of bromine for increasing amounts of chlorine resulted in violet emission with faster lifetimes, while larger proportions of bromine resulted in green emission with increased luminescence intensity. By loading FAPbBr3 nanocrystals into a PVT-based plastic scintillator matrix, we produced 1 mm-thick nanocomposite scintillators, which have brighter luminescence than the PVT-based plastic scintillator alone. While nanocomposites such as these are often opaque due to optical scattering from aggregates of the nanoparticles, we used a surface modification technique to improve transmission through the composites. A composite of FAPbBr3 nanocrystals encapsulated in inert PMMA produced even stronger luminescence, with intensity 3.8× greater than a comparative FAPbBr3/plastic scintillator composite. However, the luminescence decay time of the FAPbBr3/PMMA composite was more than 3× slower than that of the FAPbBr3/plastic scintillator composite. We also demonstrate the potential of these lead halide perovskite nanocomposite scintillators for low-cost X-ray imaging applications.
This paper reports on the performance of the inorganic scintillator caesium hafnium chloride (CHC) under exposure to the mixed radiation field of an AmBe neutron source and coupled to a silicon photomultiplier (SiPM). The neutron response is determined using the pulse shape discrimination charge comparison technique which can clearly identify both the (n,a) and (c) reactions in the material. Figures of merit for the pulse shape discrimination are presented and the quenching of the different channels is assessed through comparison to Monte Carlo simulations.
An array consisting of 12 deuterated organic liquid scintillator detectors for fast-neutron spectroscopy was designed and built at Oak Ridge National Laboratory (ORNL). This versatile array is designed for measurements with low reaction yields, such as those performed with rare isotope beams, as well as at high current DC facilities used for underground nuclear astrophysics research. Because some measurements also offer limited, or no, additional timing information, the ORNL Deuterated Spectroscopic Array (ODeSA) was optimized to utilize spectrum unfolding to extract neutron energy spectra. This array was characterized for n/ pulse shape discrimination, light response, resolution, and intrinsic efficiency by using the neutron time-of-flight tunnel at the Edwards Accelerator Laboratory at Ohio University. Results and future plans are discussed.
An array of neutron detectors based on liquid scintillator technology has been developed for nuclear reaction studies, in particular measurements with radioactive ion beams (RIBs). Reaction measurements with RIBs often involve low reaction yields and high gamma-induced backgrounds, which requires high solid angle coverage, high efficiency, and background reduction capability. The kinematics of the reaction neutrons require energy and position determination with good resolution, but preferably without a large number of readouts to minimize cost. To address these requirements, the Scintillation Array of Bars for Reaction Experiments (SABRE) consists of five 12” long, 2” diameter bar detectors filled with organic liquid scintillator and capped at both ends with individually read-out superbialkali photomultiplier tubes (PMTs). In-house purification of the scintillator mixtures resulted in improved light collection. Discussion of the liquid scintillator development and the design and commissioning of SABRE will be presented.