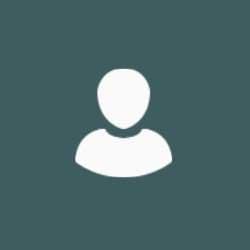
Dr Giuseppina Mariano
About
Biography
I obtained my PhD at the University of Dundee in 2018, investigating the mechanism of antibacterial toxins secreted by the Type VI Secretion System.
After a short post-doctoral experience in Sheffield in 2020, I was awarded a Wellcome Trust Sir Henry Wellcome fellowship for 4 years to investigate the mechanism of antiphage systems. In 2023, I was appointed as a Lecturer at the University of Surrey, and my research programme mainly focuses on discovering and characterising antiphage systems in Serratia marcescens. We further investigate the mobilisation and evolution of these systems within genera, and we ultimately aim to understand the ecological, evolutionary and commercial impact of novel antiphage systems.
ResearchResearch interests
Bacteriophages (phages) outnumber bacteria in the environment. This selective pressure has driven bacteria to develop many anti-viral strategies and phages to evolve counter-measures. In recent years ~170 new antiphage systems have been discovered in bacterial genomes. Understanding this arms race is revealing exciting new areas of biology, and unexpected links between phage defence and the innate immune systems of animals and plants.
My research uses comparative genomics, genetics, biochemistry, single-cell microscopy and structural biology to discover new loci involved in phage defence and define the mechanistic details of their defence pathways, including understanding how interaction with phages triggers these systems and the downstream responses that lead to immunity. I am particularly focused on antiphage systems found in Serratia marcescens (and other spp.), but the lab also works on Escherichia coli, Pseudomonas aeruginosa and Bacillus spp.
Research interests
Bacteriophages (phages) outnumber bacteria in the environment. This selective pressure has driven bacteria to develop many anti-viral strategies and phages to evolve counter-measures. In recent years ~170 new antiphage systems have been discovered in bacterial genomes. Understanding this arms race is revealing exciting new areas of biology, and unexpected links between phage defence and the innate immune systems of animals and plants.
My research uses comparative genomics, genetics, biochemistry, single-cell microscopy and structural biology to discover new loci involved in phage defence and define the mechanistic details of their defence pathways, including understanding how interaction with phages triggers these systems and the downstream responses that lead to immunity. I am particularly focused on antiphage systems found in Serratia marcescens (and other spp.), but the lab also works on Escherichia coli, Pseudomonas aeruginosa and Bacillus spp.
Supervision
Postgraduate research supervision
Investigation of a novel DNA modification pathway that promotes bacterial immunity
(PhD studentiship-on going)
Teaching
I teach on the following modules:
BMS1049-1054: Biochemistry-Understanding the chemistry of life
BMS3079:Human Microbial Diseases.
Publications
Bacteria carry numerous anti-phage systems in "defense islands" or hotspots. Recent studies have delineated the content and boundaries of these islands in various species, revealing instances of islands that encode additional factors, including antibiotic resistance genes, stress genes, type VI secretion system (T6SS)-dependent effectors, and virulence factors. Our study identifies three defense islands in the Serratia genus with a mixed cargo of anti-phage systems, virulence factors, and different types of anti-bacterial modules, revealing a widespread trend of co-accumulation that extends beyond T6SS-dependent effectors to colicins and contact-dependent inhibition systems. We further report the identification of four distinct anti-phage system/subtypes, including a previously unreported Toll/interleukin (IL)-1 receptor (TIR)-domain-containing system with population-wide immunity, and two loci co-opting a predicted T6SS-related protein for phage defense. This study enhances our understanding of the protein domains that can be co-opted for phage defense, resulting in a highly diversified anti-phage arsenal.
RN6390 is a commonly used laboratory strain of Staphylococcus aureus derived from NCTC8325. In this study, we sequenced the RN6390 genome and compared it to available genome sequences for NCTC8325. We confirmed that three prophages, Φ11, Φ12 and Φ13, which are present in NCTC8325 are absent from the genome of RN6390, consistent with the successive curing events leading to the generation of this strain. However, we noted that a separate prophage is present in RN6390 that is not found in NCTC8325. Two separate genome sequences have been deposited for the parental strain, NCTC8325. Analysis revealed several differences between these sequences, in particular, between the copy number of esaG genes, which encode immunity proteins to the type VII secreted anti-bacterial toxin, EsaD. Single nucleotide polymorphisms were also detected in ribosomal RNA genes and genes encoding microbial surface components recognizing adhesive matrix molecules (MSCRAMM) between the two NCTC8325 sequences. Comparing each NCTC8325 sequence to other strains in the RN6390 lineage confirmed that sequence assembly errors in the earlier NCTC8325 sequence are the most likely explanation for most of the differences observed.
Type VI secretion systems (T6SSs) are nanomachines widely used by bacteria to deliver toxic effector proteins directly into neighbouring cells. However, the modes of action of many effectors remain unknown. Here we report that Ssp6, an anti-bacterial effector delivered by a T6SS of the opportunistic pathogen Serratia marcescens, is a toxin that forms ion-selective pores. Ssp6 inhibits bacterial growth by causing depolarisation of the inner membrane in intoxicated cells, together with increased outer membrane permeability. Reconstruction of Ssp6 activity in vitro demonstrates that it forms cation-selective pores. A survey of bacterial genomes reveals that genes encoding Ssp6-like effectors are widespread in Enterobacteriaceae and often linked with T6SS genes. We conclude that Ssp6 and similar proteins represent a new family of T6SS-delivered anti-bacterial effectors.
Pore-forming toxins (PFTs) are widely distributed in both Gram-negative and Gram-positive bacteria. PFTs can act as virulence factors that bacteria utilise in dissemination and host colonisation or, alternatively, they can be employed to compete with rival microbes in polymicrobial niches. PFTs transition from a soluble form to become membrane-embedded by undergoing large conformational changes. Once inserted, they perforate the membrane, causing uncontrolled efflux of ions and/or nutrients and dissipating the protonmotive force (PMF). In some instances, target cells intoxicated by PFTs display additional effects as part of the cellular response to pore formation. Significant progress has been made in the mechanistic description of pore formation for the different PFTs families, but in several cases a complete understanding of pore structure remains lacking. PFTs have evolved recognition mechanisms to bind specific receptors that define their host tropism, although this can be remarkably diverse even within the same family. Here we summarise the salient features of PFTs and highlight where additional research is necessary to fully understand the mechanism of pore formation by members of this diverse group of protein toxins.
The Type VI secretion system (T6SS) is widely used by bacterial pathogens as an effective weapon against bacterial competitors and is also deployed against host eukaryotic cells in some cases. It is a contractile nanomachine which delivers toxic effector proteins directly into target cells by dynamic cycles of assembly and firing. Bacterial cells adopt distinct post-translational regulatory strategies for deployment of the T6SS. 'Defensive' T6SSs assemble and fire in response to incoming attacks from aggressive neighbouring cells, and can utilise the Threonine Protein Phosphorylation (TPP) regulatory pathway to achieve this control. However, many T6SSs are 'offensive', firing at all-comers without the need for incoming attack or other cell contact-dependent signal. Post-translational control of the offensive mode has been less well defined but can utilise components of the same TPP pathway. Here, we used the anti-bacterial T6SS of Serratia marcescens to elucidate post-translational regulation of offensive T6SS deployment, using single-cell microscopy and genetic analyses. We show that the integration of the TPP pathway with the negative regulator TagF to control core T6SS machine assembly is conserved between offensive and defensive T6SSs. Signal-dependent PpkA-mediated phosphorylation of Fha is required to overcome inhibition of membrane complex assembly by TagF, whilst PppA-mediated dephosphorylation promotes spatial reorientation and efficient killing. In contrast, the upstream input of the TPP pathway defines regulatory strategy, with a new periplasmic regulator, RtkS, shown to interact with the PpkA kinase in S. marcescens. We propose a model whereby the opposing actions of the TPP pathway and TagF impose a delay on T6SS re-assembly after firing, providing an opportunity for spatial re-orientation of the T6SS in order to maximise the efficiency of competitor cell targeting. Our findings provide a better understanding of how bacterial cells deploy competitive weapons effectively, with implications for the structure and dynamics of varied polymicrobial communities.
The type VII secretion system (T7SS) is found in many Gram--positive firmicutes and secretes protein toxins that mediate bacterial antagonism. Two T7SS toxins have been identified in Staphylococcus aureus, EsaD a nuclease toxin that is counteracted by the EsaG immunity protein, and TspA, which has membrane depolarising activity and is neutralised by TsaI. Both toxins are polymorphic, and strings of non--identical esaG and tsaI immunity genes are encoded in all S. aureus strains. To investigate the evolution of esaG repertoires, we analysed the sequences of the tandem esaG genes and their encoded proteins. We identified three blocks of high sequence similarity shared by all esaG genes and identified evidence of extensive recombination events between esaG paralogues facilitated through these conserved sequence blocks. Recombination between these blocks accounts for loss and expansion of esaG genes in S. aureus genomes and we identified evidence of such events during evolution of strains in clonal complex 8. TipC, an immunity protein for the TelC lipid II phosphatase toxin secreted by the streptococcal T7SS, is also encoded by multiple gene paralogues. Two blocks of high sequence similarity locate to the 5' and 3' end of tipC genes, and we found strong evidence for recombination between tipC paralogues encoded by Streptococcus mitis BCC08. By contrast, we found only a single homology block across tsaI genes, and little evidence for intergenic recombination within this gene family. We conclude that homologous recombination is one of the drivers for the evolution of T7SS immunity gene clusters.
Severe acute respiratory syndrome coronavirus 2 (SARS-CoV-2) has rapidly spread in humans in almost every country, causing the disease COVID-19. Since the start of the COVID-19 pandemic, research efforts have been strongly directed towards obtaining a full understanding of the biology of the viral infection, in order to develop a vaccine and therapeutic approaches. In particular, structural studies have allowed to comprehend the molecular basis underlying the role of many of the SARS-CoV-2 proteins, and to make rapid progress towards treatment and preventive therapeutics. Despite the great advances that have been provided by these studies, many knowledge gaps on the biology and molecular basis of SARS-CoV-2 infection still remain. Filling these gaps will be the key to tackle this pandemic, through development of effective treatments and specific vaccination strategies.
The bacterial flagellum is a complex, self-assembling macromolecular machine that powers bacterial motility. It plays diverse roles in bacterial virulence, including aiding in colonization and dissemination during infection. The flagellum consists of a filamentous structure protruding from the cell, and of the basal body, a large assembly that spans the cell envelope. The basal body is comprised of over 20 different proteins forming several concentric ring structures, termed the M- S- L- P- and C-rings, respectively. In particular, the MS rings are formed by a single protein FliF, which consists of two trans-membrane helices anchoring it to the inner membrane and surrounding a large periplasmic domain. Assembly of the MS ring, through oligomerization of FliF, is one of the first steps of basal body assembly. Previous computational analysis had shown that the periplasmic region of FliF consists of three structurally similar domains, termed Ring-Building Motif (RBM)1, RBM2, and RBM3. The structure of the MS-ring has been reported recently, and unexpectedly shown that these three domains adopt different symmetries, with RBM3 having a 34-mer stoichiometry, while RBM2 adopts two distinct positions in the complex, including a 23-mer ring. This observation raises some important question on the assembly of the MS ring, and the formation of this symmetry mismatch within a single protein. In this study, we analyze the oligomerization of the individual RBM domains in isolation, in the Salmonella enterica serovar Typhimurium FliF ortholog. We demonstrate that the periplasmic domain of FliF assembles into the MS ring, in the absence of the trans-membrane helices. We also report that the RBM2 and RBM3 domains oligomerize into ring structures, but not RBM1. Intriguingly, we observe that a construct encompassing RBM1 and RBM2 is monomeric, suggesting that RBM1 interacts with RBM2, and inhibits its oligomerization. However, this inhibition is lifted by the addition of RBM3. Collectively, this data suggest a mechanism for the controlled assembly of the MS ring.
Incorporation of disulfide bonds into proteins can be critical for function or stability. In bacterial cells, the periplasmic enzyme DsbA is responsible for disulfide incorporation into many extra-cytoplasmic proteins. The type VI secretion system (T6SS) is a widely occurring nanomachine that delivers toxic effector proteins directly into rival bacterial cells, playing a key role in inter-bacterial competition. We report that two redundant DsbA proteins are required for virulence and for proper deployment of the T6SS in the opportunistic pathogen Serratia marcescens, with several T6SS components being subject to the action of DsbA in secreting cells. Importantly, we demonstrate that DsbA also plays a critical role in recipient target cells, being required for the toxicity of certain incoming effector proteins. Thus we reveal that target cell functions can be hijacked by T6SS effectors for effector activation, adding a further level of complexity to the T6SS-mediated inter-bacterial interactions which define varied microbial communities.
Competitive bacteria-bacteriophage interactions have resulted in the evolution of a plethora of bacterial defense systems preventing phage propagation. In recent years, computational and bioinformatic approaches have underpinned the discovery of numerous novel bacterial defense systems. Anti-phage systems are frequently encoded together in genomic loci termed defense islands. Here we report the identification and characterisation of a novel anti-phage system, that we have termed Shield, which forms part of the Pseudomonas defensive arsenal. The Shield system comprises the core component ShdA, a membrane-bound protein harboring an RmuC domain. Heterologous production of ShdA alone is sufficient to mediate bacterial immunity against several phages. We demonstrate that Shield and ShdA confer population-level immunity and that they can also decrease transformation efficiency. We further show that ShdA homologues can degrade DNA in vitro and, when expressed in a heterologous host, can alter the organisation of the host chromosomal DNA. Use of comparative genomic approaches identified how Shield can be divided into four subtypes, three of which contain additional components that in some cases can negatively affect the activity of ShdA and/or provide additional lines of phage defense. Collectively, our results identify a new player within the Pseudomonas bacterial immunity arsenal that displays a novel mechanism of protection, and reveals a role for RmuC domains in phage defense.
Additional publications
- Kelly,A, Went, S.C., Mariano, G., Shaw, L.P., Picton, D.M., Duffnera, S.J.; et al., (2023). Diverse Durham collection phages demonstrate complex BREX defence responses. AEV, In Press
- Mariano, G.*, and Blower, T.R. (2023). Conserved domains can be found across distinct phage defence systems. Mol Microbiol. 10.1111/mmi.15047.
- Ulhuq, F. R., and Mariano, G*. (2022). Bacterial pore-forming toxins. Microbiology, 168. *Lead and corresponding author
- Garrett, S.R., Mariano, G., and Palmer, T. (2022) Genomic analysis of Staphylococcus aureus strain RN6390 progenitor strains. Access Microbiology
- Garrett, S.R., Mariano, G., Dicks, J., and Palmer, T. (2022). Homologous recombination between tandem paralogues drives evolution of a subset of type VII secretion system immunity genes in firmicute bacteria. Microbial Genomics 8, 000868. 10.1099/mgen.0.000868.
- Mariano, G., Faba-Rodriguez, R., Bui, S., Zhao, W., Ross, J., Tzokov, S. B., et al. (2022). Oligomerization of the FliF Domains Suggests a Coordinated Assembly of the Bacterial Flagellum MS Ring. Front. Microbiol. 0. doi: 10.3389/fmicb.2021.781960.
- Mariano, G*., Farthing, R. J., Lale-Farjat, S. L. M., and Bergeron, J. R. C. (2020). Structural Characterization of SARS-CoV-2: Where We Are, and Where We Need to Be. Front Mol Biosci 7, 605236. doi:10.3389/fmolb.2020.605236. * Co-corresponding author
- Mariano, G., Trunk, K., Williams, D. J., Monlezun, L., Strahl, H., Pitt, S. J., & Coulthurst, S. J. (2019). A family of Type VI secretion system effector proteins that form ion-selective pores. Nature Communications, 10(1), 1-15. (Chosen for Nature Review Microbiology Research Highlights)
- Mariano, G., Monlezun, L., & Coulthurst, S. J. (2018). Dual Role for DsbA in Attacking and Targeted Bacterial Cells during Type VI Secretion System-Mediated Competition. Cell Reports, 22(3), 774-785. (This publication was recommended by F1000 society).
- Ostrowski, A., Cianfanelli, F.R., Porter, M., Mariano, G., Peltier, J., Wong, J.J., Swedlow, J.R., Trost, M., and Coulthurst, S.J. (2018). Killing with proficiency: Integrated post-translational regulation of an offensive Type VI secretion system. PLoS Pathog. 14, e1007230.