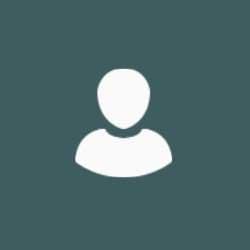
Dr Dimitrios Charaklias
Publications
Transparent thermal heaters based on metallic networks have gained considerable attention in the last few years as a result of their superior response time, low sheet resistance and low cost of manufacturing. To increase the mechanical stability and reliability of the thermal heater, it is desirable to embed the metallic network in some form of matrix. Embedding the network however, changes the nature of thermal conduction making both in-plane and out-of-plane thermal conduction important for ensuring reliability and uniform thermal distribution. The performance of embedded thermal heaters is also significantly influenced by the geometry of metallic network, both in terms of optical transparency and thermal performance. In this paper, we have developed a coupled electro-thermal model and an electromagnetic model to investigate the properties of an embedded metallic mesh in a polymer matrix. IR thermal imaging and UV spectrophotometer have been used to quantify thermal transport and transparency in the system and to verify the performance of FE models. A systematic study is then performed to assess the role of network topology both on in-plane and out-of-plane thermal distribution and optical performance. According to numerical analysis, a structure-property relationship has been established which could provide desirable network configurations to optimize performance.
Thermoplastic softening is one of the most desirable de-stiffening methods because of its reversibility, scalability, and applicability in many of current multi-layered structures without compromising structural performance. Despite the advantages, long activation times and high activation power requirements are generally considered as the main drawbacks for this method which can potentially limit its application in scenarios where fast de-stiffening is required. The aim of this study is to identify the key design requirements of heating element to minimise the de-stiffening response time using thermoplastic softening while maximising transparency. The focus of this study is on multilayer transparent structures, with low heating element content. A systematic investigation, including experimental and numerical investigation, is performed to study the effect of the fill factor and the heating element’s length scale on the response time of de-stiffening. Melting of the polymer and melting or electrical breakdown of the heating element are observed as practical limitations and are introduced as constraints to the design maps. The fill factor is found to have considerable influence on improving the response time, especially at low fill factors (i.e. below 10%). For the material combinations investigated here, the design maps show that heating elements with wire diameters up to 7 μm, at maximum transparency of 2% fill factor and up to 12 μm at 20% fill factor can achieve sub-second response times for temperature increase of 30⁰C. This new understanding will accelerate the technology readiness level of active structural control technology to be used in the future multi-functional and smart structures with a wide range of application in robotics, shape morphing, active damping, and active impact protection.
In constant pursuit of lightweight, efficient, and resilient structures, the focus to Multi-Functional Structure (MSF) has increasing grown over the past decade. The MFSs combine load-bearing capacity with other functionalities such as thermal/electrical conductivity or insulation, electromagnetic shielding, energy storage, self-healing, stiffness modulation, or any combination of them. In constant pursuit of lightweight, efficient, and resilient structures, attention to Multi-Functional Structure (MSF) has increasing grown over the last decade. The MFSs often combine load-bearing capacity with other functionalities such as thermal/electrical conductivity or insulation, electromagnetic shielding, energy storage, self-healing, stiffness modulation, or any combination of them. Often constituted of hybrid materials, MFSs employ stimuli-responsive materials, allowing them to adapt to external factors like heat, pressure, electrical current, magnetic or electrical fields, moisture, pH levels, or light. Amongst various methods, thermal softening in thermoplastics is one of the most desirable de-stiffening methods because of its reversibility, scalability, and applicability in many of current multi-layered structures without compromising structural performance. However, the reliance on delivering thermal energy introduces challenges such as prolonged activation times and high-power requirements, limiting its application in scenarios requiring rapid de-stiffening, such as impact protection. This paper systematically investigates the parameters influencing the response time of de-stiffening, focusing on characteristics such as the size of the heating element and the volume, as well as the thermal properties of the material to be heated. We employed three types of heating elements embedded in multi-layered structures, namely wires, structured metallic meshes, and random metallic meshes (refer to Figure 1). Our findings demonstrate that achieving a very fast heating rate (45°C·s-1) is feasible using random metallic meshes under 4.8 V excitation. Figure 1: Embedding various types of heating element in multi-layered structures.
The majority of natural organisms interact with their environments with a degree of mechanical adaptability that allows them to carry out a variety of tasks and adapt to changing circumstances. Human-made structures, however, lack this versatility and are normally designed to fulfill a certain load-carrying requirement. This causes limitations in performance, efficiency, and safety. The aim of this article is to present rapid de-stiffening in the response of conventional structures, without compromising the load-bearing capacity. This has been achieved by developing an active interface using an interconnected nanostructured metallic network. A very fast heating rate with an average of approximate to 45 degrees C s-1 under 4.8 V excitation while retaining transparency of 67% is demonstrated. The embedded metallic network in a thermoplastic matrix has been deployed as an active interface, in a conventional transparent multilayered structure. Upon activation, it provides a rapid (i.e., 2 s after activation) mechanical de-stiffening capability. The results from finite element modeling have been found to be in good agreements with those from experiments. The rapid reversible stiffness tuning demonstrated here can be implemented in variety of multilayered structures with a wide range of applications in robotics, morphing and deployable structures, active damping, and active impact safety systems. Herein, a transparent active interface is presented, which is manufactured using a template-based technique and is capable of very fast heating rates (approximate to 45 degrees C s-1 under 4.8 V excitation). The proposed interface can be utilized in many conventional multilayer structures and have great potentials for improving impact safety in pedestrian crashing or in human-robot interaction by fast de-stiffening of structures.image (c) 2024 WILEY-VCH GmbH