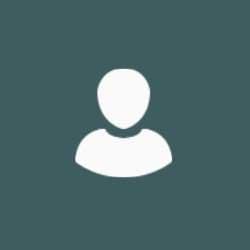
Davide Maselli
Publications
Dengue is the most prevalent arthropod-borne viral disease affecting humans, with severe dengue typified by potentially fatal microvascular leakage and hypovolaemic shock. Blood vessels of the microvasculature are composed of a tubular structure of endothelial cells ensheathed by perivascular cells (pericytes). Pericytes support endothelial cell barrier formation and maintenance through paracrine and contact-mediated signalling, and are critical to microvascular integrity. Pericyte dysfunction has been linked to vascular leakage in noncommunicable pathologies such as diabetic retinopathy, but has never been linked to infection-related vascular leakage. Dengue vascular leakage has been shown to result in part from the direct action of the secreted dengue virus (DENV) non-structural protein NS1 on endothelial cells. Using primary human vascular cells, we show here that NS1 also causes pericyte dysfunction, and that NS1-induced endothelial hyperpermeability is more pronounced in the presence of pericytes. Notably, NS1 specifically disrupted the ability of pericytes to support endothelial cell function in a 3D microvascular assay, with no effect on pericyte viability or physiology. These effects are mediated at least in part through contact-independent paracrine signals involved in endothelial barrier maintenance by pericytes. We therefore identify a role for pericytes in amplifying NS1-induced microvascular hyperpermeability in severe dengue, and thus show that pericytes can play a critical role in the aetiology of an infectious vascular leakage syndrome. These findings open new avenues of research for the development of drugs and diagnostic assays for combating infection-induced vascular leakage, such as severe dengue.
Routine cardiovascular interventions such as balloon angioplasty, result in vascular activation and remodeling, often requiring re-interventions. 2D in vitro culture models and small animal experiments have enabled the discovery of important molecular and cellular pathways involved in this process, however the clinical translation of these results is often underwhelming. There is a critical need for an ex vivo model representative of the human vascular physiology and encompassing the complexity of the vascular wall and the physical forces regulating its function. Vascular bioreactors for ex vivo culture of large vessels are viable alternatives, but their custom-made design and insufficient characterization often hinders the reproducibility of the experiments. The objective of the study was to design and validate a novel 3D printed cost-efficient and versatile perfusion system, capable of sustaining the viability and functionality of large porcine arteries for 7 days and enabling monitoring of post-injury remodeling. MultiJet Fusion 3D printing technology was used to engineer the EasyFlow insert, converting a conventional 50 ml centrifuge tube into a mini bioreactor. Porcine carotid arteries either left untreated or injured with a conventional angioplasty balloon, were cultured under pulsatile flow for up to 7 days. Pressure, heart rate, medium viscosity and shear conditions were adjusted to represent the typical arterial physiology. Tissue viability, cell activation and matrix remodeling were analyzed by immunohistochemistry, and vascular function was monitored by duplex ultrasound. Physiological blood flow conditions in the EasyFlow bioreactor preserved endothelial coverage and smooth muscle organization and extracellular matrix structure in the vessel wall, as compared to static culture. Injured arteries presented hallmarks of early remodeling, such as intimal denudation, smooth muscle cell disarray and media/adventitia activation. Duplex ultrasound confirmed physiological hemodynamic conditions, dose-dependent vasodilator response to nitroglycerin in untreated vessels and impaired dilator response in angioplastied vessels. We here validate a low-cost, robust and reproducible system to study large blood vessels physiopathology, laying the basis for future investigations into the pathological remodeling of blood vessels and creating a platform to test novel therapies and devices ex vivo in a patient relevant system. Competing Interest Statement The authors have declared no competing interest.
The epicardium constitutes an untapped reservoir for cardiac regeneration. Upon heart injury, the adult epicardium re-activates, leading to epithelial-to-mesenchymal transition (EMT), migration and differentiation. While interesting mechanistic and therapeutic findings arose from lower vertebrates and rodent models, the introduction of an experimental system representative of large mammals would undoubtedly facilitate translational advancements. Here, we apply innovative protocols to obtain living 3D organotypic epicardial slices from porcine hearts, encompassing the epicardial/myocardial interface. In culture, our slices preserve the in vivo architecture and functionality, presenting a continuous epicardium overlaying a healthy and connected myocardium. Upon thymosin β4 treatment of the slices, the epicardial cells become activated upregulating epicardial and EMT genes, resulting in epicardial cell mobilization and differentiation into epicardial-derived mesenchymal cells. Our 3D organotypic model enables to investigate the reparative potential of the adult epicardium, offering an advanced tool to explore ex vivo the complex 3D interactions occurring within the native heart environment
The epicardium constitutes an untapped reservoir for cardiac regeneration. Upon heart injury, the adult epicardium re-activates, leading to epithelial-to-mesenchymal transition (EMT), migration, and differentiation. While interesting mechanistic and therapeutic findings arose from lower vertebrates and rodent models, the introduction of an experimental system representative of large mammals would undoubtedly facilitate translational advancements. Here, we apply innovative protocols to obtain living 3D organotypic epicardial slices from porcine hearts, encompassing the epicardial/myocardial interface. In culture, our slices preserve the in vivo architecture and functionality, presenting a continuous epicardium overlaying a healthy and connected myocardium. Upon thymosin β4 treatment of the slices, the epicardial cells become activated, upregulating epicardial and EMT genes, resulting in epicardial cell mobilization and differentiation into epicardial-derived mesenchymal cells. Our 3D organotypic model enables to investigate the reparative potential of the adult epicardium, offering an advanced tool to explore ex vivo the complex 3D interactions occurring within the native heart environment.
Vascular disease forms the basis of most cardiovascular diseases (CVDs), which remain the primary cause of mortality and morbidity worldwide. Efficacious surgical and pharmacological interventions to prevent and treat vascular disease are urgently needed. In part, the shortage of translational models limits the understanding of the cellular and molecular processes involved in vascular disease. Ex vivo perfusion culture bioreactors provide an ideal platform for the study of large animal vessels (including humans) in a controlled dynamic environment, combining the ease of in vitro culture and the complexity of the live tissue. Most bioreactors are, however, custom manufactured and therefore difficult to adopt, limiting the reproducibility of the results. This paper presents a 3D printed system that can be easily produced and applied in any biological lab, and provides a detailed protocol for its setup, enabling users operation. This innovative and reproducible ex vivo perfusion culture system enables the culture of blood vessels for up to 7 days in physiological conditions. We expect that adopting a standardized perfusion bioreactor will support a better understanding of physiological and pathological processes in large animal blood vessels and accelerate the discovery of new therapeutics.
Routine interventions such as balloon angioplasty, result in vascular activation and remodeling, often requiring re-intervention. 2D in vitro models and small animal experiments have enabled the discovery of important mechanisms involved in this process, however the clinical translation is often underwhelming. There is a critical need for an ex vivo model representative of the human vascular physiology and encompassing the complexity of the vascular wall and the physical forces regulating its function. Vascular bioreactors for ex vivo culture of large vessels are viable alternatives, but their custom-made design and insufficient characterization often hinders the reproducibility of the experiments. The objective of the study was to design and validate a novel 3D printed cost-efficient and versatile perfusion system, capable of sustaining the viability and functionality of large porcine arteries for 7 days and enabling early post-injury evaluations. MultiJet Fusion 3D printing was used to engineer the EasyFlow insert, converting a conventional 50 ml centrifuge tube into a mini bioreactor. Porcine carotid arteries either left untreated or injured with an angioplasty balloon, were cultured under pulsatile flow for up to 7 days. Pressure, heart rate, medium viscosity and shear conditions were adjusted to resemble arterial in vivo hemodynamics. Tissue viability, cell activation and matrix remodeling were analyzed by immunohistochemistry, and vascular function was monitored by duplex ultrasound. Culture conditions in the EasyFlow bioreactor preserved endothelial coverage and smooth muscle organization and extracellular matrix structure in the vessel wall, as compared to static culture. Injured arteries presented hallmarks of early remodeling, such as intimal denudation, smooth muscle cell disarray and media/adventitia activation in flow culture. Duplex ultrasound confirmed continuous pulsatile blood flow conditions, dose-dependent vasodilator response to nitroglycerin in untreated vessels and impaired dilator response in angioplastied vessels. The scope of this work is to validate a low-cost, robust and reproducible system to explore the culture of native and injured large arteries under pulsatile flow. While the study of vascular pathology is beyond the scope of the present paper, our system enables future investigations and provides a platform to test novel therapies and devices ex vivo, in a patient relevant system.
The epicardium has recently gained interest in the cardiovascular field due to its capacity to support heart regeneration after ischemic injury. Models to study the epicardium of large animals in vitro are limited and mainly based on epicardial cell isolation/differentiation from stem cells, followed by 2D cells culture. In this method paper, we describe the procedure to obtain and culture 3D organotypic heart slices presenting an intact epicardium, as a novel model to study the epicardial physiology and activation. Epicardial slices are obtained from porcine hearts using a high-precision vibratome and retain a healthy epicardial layer embedded in its native extracellular environment and connected with other cardiac cells (cardiomyocytes, fibroblasts, vascular cells etc.). Epicardial slices can be cultured for 72 h, providing an ideal model for studying the epicardium physiology or perform pharmacological interventions/gene therapy approaches. We also report on methods to assesses the viability and composition of the epicardial slices, and evaluate their architecture in 3D through tissue decoloration. Finally, we present a potential application for a nanomaterial-based gene transfer method for tracking of epicardial cells within the slice. Crucially, given the similarity in morphology and physiology of porcine heart with its human counterpart, our system provides a platform for translational research while providing a clinically relevant and ethical alternative to the use of small animals in this type of research.