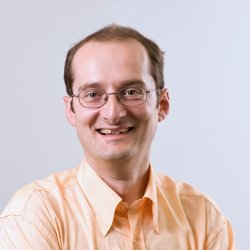
Professor Aldo Sorniotti
ResearchResearch interests
- Vehicle dynamics and simulation
- Vehicle dynamics control
- Vehicle chassis
- Transmission systems for fully electric vehicles
- Hybrid electric vehicles.
Research interests
- Vehicle dynamics and simulation
- Vehicle dynamics control
- Vehicle chassis
- Transmission systems for fully electric vehicles
- Hybrid electric vehicles.
Teaching
ENG3162 Group Design Project (Formula Student)
ENG2119 Powertrain Design and Chassis Engineering
ENG3170 Modern Vehicle Systems Design
Multi-Disciplinary Design Project
Publications
This paper presents a novel unscented Kalman filter (UKF) implementation with adaptive covariance matrices (ACMs), to accurately estimate the longitudinal and lateral components of vehicle velocity, and thus the sideslip angle, tire slip angles, and tire slip ratios, also in extreme driving conditions, including tyre–road friction variations. The adaptation strategies are implemented on both the process noise and measurement noise covariances. The resulting UKF ACM is compared against a well-tuned baseline UKF with fixed covariances. Experimental test results in high tyre–road friction conditions show the good performance of both filters, with only a very marginal benefit of the ACM version. However, the simulated extreme tests in variable and low-friction conditions highlight the superior performance and robustness provided by the adaptation mechanism.
Active and semi-active suspensions for passenger cars traditionally enhance comfort through body control, and vehicle handling by reducing the tyre load variations induced by road irregularities. Active suspensions can also be designed to track a desired yaw rate profile through the control of the lateral load transfer distribution between the front and rear axles. This paper considers an integrated system including semi-active and active suspension actuation to control the yaw, roll, pitch and heave dynamics excited by the driving actions. To this purpose, two novel real-time-capable implicit nonlinear model predictive control (NMPC) formulations, excluding and including cost function weight adaptation, are proposed and compared with the passive vehicle, and the controlled vehicle with two combinations of skyhook and active roll control, the first based on a pseudoinverse decoupling transformation for obtaining the damping force contributions, and the second using an inverse formulation. The algorithms are assessed through an experimentally validated simulation model, along manoeuvres corresponding to sub-limit and limit handling operation, to analyse the trade-off between body motion reduction and cornering response enhancement. The results show that the adaptable NMPC configuration provides the best performance in all scenarios, also for significant variations of the main vehicle and tyre parameters.
In passenger cars, the ride characteristics are fundamental to the driver and passenger engagement, as they define the comfort and road holding performance. Therefore, the methods and tools to assess ride quality are of significant interest to the vehicle dynamics specialists, and are an important part of the internal know-how of each car maker and Tier 1 supplier, which is often kept confidential. Unfortunately, the available literature does not include a comprehensive survey on the evaluation of the objective and subjective aspects related to ride, and their correlation. This review targets the gap, and deals with: (i) the available tools and techniques to objectively assess primary and secondary ride, including typical manoeuvres, road profiles, required vehicle instrumentation, and key performance indicators (KPIs); (ii) the subjective attributes and their categorisation; (iii) the approaches and mathematical models to correlate the objective KPIs with the subjective evaluation; and (iv) future trends. The know-how of the authors on the ride assessment of high-performance passenger cars will also be used to cover the aspects that are currently overlooked by the available literature and standards. In summary, the manuscript provides the interested reader with useful guidance on the procedures to perform ride quality analyses.
A recently growing literature discusses the topics of direct yaw moment control based on model predictive control (MPC), and energy-efficient torque-vectoring (TV) for electric vehicles with multiple powertrains. To reduce energy consumption, the available TV studies focus on the control allocation layer, which calculates the individual wheel torque levels to generate the total reference longitudinal force and direct yaw moment, specified by higher level algorithms to provide the desired longitudinal and lateral vehicle dynamics. In fact, with a system of redundant actuators, the vehicle-level objectives can be achieved by distributing the individual control actions to minimize an optimality criterion, e.g., based on the reduction of different power loss contributions. However, preliminary simulation and experimental studies - not using MPC - show that further important energy savings are possible through the appropriate design of the reference yaw rate. This paper presents a nonlinear model predictive control (NMPC) implementation for energy-efficient TV, which is based on the concurrent optimization of the reference yaw rate and wheel torque allocation. The NMPC cost function weights are varied through a fuzzy logic algorithm to adaptively prioritize vehicle dynamics or energy efficiency, depending on the driving conditions. The results show that the adaptive NMPC configuration allows stable cornering performance with lower energy consumption than a benchmarking fuzzy logic TV controller using an energy-efficient control allocation layer.
In passenger cars active suspensions have been traditionally used to enhance comfort through body control, and handling through the reduction of the tyre load variations induced by road irregularities. However, active suspensions can also be designed to track a desired yaw rate profile through the control of the anti-roll moment distribution between the front and rear axles. The effect of the anti-roll moment distribution relates to the nonlinearity of tyre behaviour, which is difficult to capture in the linearised vehicle models normally used for control design. Hence, the tuning of anti-roll moment distribution controllers is usually based on heuristics. This paper includes an analysis of the effect of the lateral load transfer on the lateral axle force and cornering stiffness. A linearised axle force formulation is presented, and compared with a formulation from the literature, based on a quadratic relationship between cornering stiffness and load transfer. Multiple linearised vehicle models for control design are assessed in the frequency domain, and the respective controllers are tuned through optimisation routines. Simulation results from a nonlinear vehicle model are discussed to analyse the performance of the controllers, and show the importance of employing accurate models of the lateral load transfer effect during control design.
This study discusses vehicle stability control based on explicit nonlinear model predictive control (NMPC) and investigates the influence of prediction model fidelity on controller performance. The explicit solutions are generated through an algorithm using multiparametric quadratic programming (mp-QP) approximations of the multiparametric nonlinear programming (mp-NLP) problems. Controllers with different prediction models are assessed through objective indicators in sine-with-dwell tests. The analysis considers the following prediction model features: 1) nonlinear lateral tire forces as functions of slip angles, which are essential for the operation of the stability controller at the limit of handling. Moreover, a simple nonlinear tire force model with saturation is shown to be an effective alternative to a more complex model based on a simplified version of the Magic Formula; 2) longitudinal and lateral load transfers, playing a crucial role in the accurate prediction of the lateral tire forces and their yaw moment contributions; 3) coupling between longitudinal and lateral tire forces, which has a significant influence on the front-to-rear distribution of the braking forces generated by the controller; and 4) nonlinear peak and stiffness factors of the tire model, with visible yet negligible effects on the results.
Integrated Chassis Control (ICC) is one of the most appealing subjects for vehicle dynamics specialists and researchers, due to the increasing number of chassis actuators of modern human-driven and automated cars. ICC ensures that the potential of the available actuators is systematically exploited, by overcoming the individual limitations, and solving conflicts and redundancies, which results into enhanced vehicle performance, ride comfort and safety. This paper is a literature review on ICC, and focuses on the topics that are left uncovered by the most recent surveys on the subject, or that are dealt with only by old surveys, namely: a) the systematic categorisation of the available ICC architectures, with the critical analysis of their strengths and weaknesses; b) the latest ICC approaches, which are becoming feasible with modern automotive microcontrollers; c) the driving performance requirements; and d) the procedures to objectively evaluate ICC performance. The manuscript aids the interested reader in the choice of the most appropriate ICC method for the specific requirements, and concludes with the recent developments and future trends.
Electric Vehicles (EVs) with multiple motors permit to design the steady-state cornering response by imposing reference understeer characteristics according to expected vehicle handling quality targets. To this aim a direct yaw moment is generated by assigning different torque demands to the left and right vehicle sides. The reference understeer characteristic has an impact on the drivetrain input power as well. In parallel, a Control Allocation (CA) strategy can be employed to achieve an energy-efficient wheel torque distribution generating the reference yaw moment and wheel torque. To the knowledge of the authors, for the first time this paper experimentally compares and critically analyses the potential energy efficiency benefits achievable through the appropriate set-up of the reference understeer characteristics and wheel torque CA. Interestingly, the experiments on a four wheel-drive EV demonstrator show that higher energy savings can be obtained through the appropriate tuning of the reference cornering response rather than with an energy efficient CA.
A significant body of literature discusses direct yaw moment controllers for vehicle stability control and torque-vectoring (TV), based on model predictive control. However, the available references lack an analysis of the effect of including or excluding the wheel dynamics in the prediction model in combined longitudinal and lateral acceleration conditions, which is related to the control system architecture. In fact, in the first case, the controller can also fulfill the wheel slip control function, according to a centralized architecture, while in the second case, the tire slip limitation has to be implemented externally, in a multi-layer approach. This study addresses the identified gap by proposing and comparing-through simulations with a high-fidelity vehicle model-centralized and multilayer real-time implementable architectures using nonlinear model predictive control (NMPC) for the TV and traction control (TC) of an electric vehicle with front in-wheel motors. An optimization routine calibrates the main controller parameters, to ensure fairness in the comparison during extreme accelerating-while-cornering maneuvers with transient steering inputs. The results show that the real-time implementable multi-layer architecture with wheel dynamics in the NMPC prediction model, and considering the externally generated TC torque reduction in the TV layer, provides equivalent performance to a centralized set-up.
The first part of the article describes the targets related to the design of an Active Roll Control (ARC) system, based on the hydraulic actuation of the anti-roll bars of an automobile. Then the basic static and dynamic design principles of the system are commented upon in detail. The second part of the article presents the hardware-in-the-loop test bench implemented to evaluate the designed system. In the end, the main experimental results are summarized and discussed from the point of view of the integration of ARC with electronic stability program.
Because of the introduction of progressively more restrictive regulations that aim to reduce fuel consumption and CO2 emissions, the automotive industry is focusing its efforts on environmentally friendly passenger cars. In particular, different electrification roadmaps are pursued by the individual car makers, and electrified drivetrain layouts are progressively introduced into the market. This process involves all vehicle segments, including high performance passenger cars. In this context, this study presents a novel hybridized automated manual transmission (HAMT) for cars with high power characteristics. The HAMT includes six gear ratios associated with the internal combustion engine, and two gear ratios associated with an electric motor. The energy efficiency of the HAMT is measured on a drivetrain test rig, and compared with that of an equivalent dual clutch transmission (DCT). Simulation results based on the experimentally measured efficiency maps show the significant energy consumption reduction of the HAMT in its internal combustion engine mode, with respect to the DCT.
© IFAC.Using Lyapunov theory, Pontryagin's minimum principle, and affine quadratic stability, a novel robust optimal control strategy is developed for active suspension systems to enhance vehicle ride comfort and handling performance. The controller has a simple structure, making its suitable for real-time implementation. The required sensor configuration includes a six-axis IMU and four LVDTs. The proposed controller is suitable for on-road commercial vehicles where ride comfort over bump disturbances and handling performance are the most concerns. The effectiveness of the controller is verified through simulation results using IPG CarMaker software.
This paper presents a traction controller for combined driving and cornering conditions, based on explicit nonlinear model predictive control. The prediction model includes a nonlinear tire force model using a simplified version of the Pacejka Magic Formula, incorporating the effect of combined longitudinal and lateral slips. Simulations of a front-wheel-drive electric vehicle with multiple motors highlight the benefits of the proposed formulation with respect to a controller with a tire model for pure longitudinal slip. Objective performance indicators provide a performance assessment in traction control scenarios.
Road irregularities induce vertical and longitudinal vibrations of the sprung and unsprung masses, which affect vehicle comfort. While the vertical dynamics and related compensation techniques are extensively covered by the suspension control literature, the longitudinal dynamics on uneven road surfaces are less frequently addressed, and are significantly influenced by the tires and suspension systems. The relatively slow response of internal combustion engines does not allow any form of active compensation of the effect of road irregularities. However, in-wheel electric powertrains, in conjunction with pre-emptive control based on the information on the road profile ahead, have some potential for effective compensation, which, however, has not been explored yet. This paper presents a proof-of-concept nonlinear model predictive control (NMPC) implementation based on road preview, which is preliminarily assessed with a simulation model of an all-wheel drive electric vehicle with in-wheel motors, including a realistic tire model for ride comfort simulation. The major improvement brought by the proposed road preview controller is evaluated through objective performance indicators along multiple maneuvers, and is confirmed by the comparison with two benchmarking feedback controllers from the literature.
A method for controlling a power assisted propulsion system in a motor vehicle so as to perform a transition from a lower gear to a higher gear without interrupting a driving torque applied to driving wheels; the propulsion system displays an internal combustion engine provided with a drive shaft and a power assisted transmission comprising in turn: a power assisted mechanical gearbox provided with a primary shaft connectable to the drive shaft and a secondary shaft connected to a transmission shaft which transmits motion to the driving wheels; a power assisted clutch interposed between the drive shaft and the primary shaft of the gearbox to connect and disconnect the drive shaft to the primary shaft of the gearbox; a power assisted brake; and an epicycloidal gear having three rotating elements: a first rotating element connected to the drive shaft, a second rotating element connected to the secondary shaft of the gearbox, and a third rotating element connected to the brake.
The paper describes the advantages due to the adoption of multi-speed transmission systems within fully electric vehicles. In particular, the article compares a conventional single-speed transmission layout, a 2-speed layout based on a novel gearbox architecture capable of seamless gearshifts, and a Continuously Variable Transmission layout. The selection of the optimal gear ratios for the 2-speed system has been based on an optimization procedure, taking into account the efficiency characteristics of the components of the whole vehicle powertrain. The control system for the Continuously Variable Transmission system has been designed with the aim of maximizing the efficiency of the operating points of the electric motor. The results show that there is a significant advantage in adopting a 2-speed transmission system over the single-speed layout, and, despite a reduction in motor losses, the typically lower efficiency characteristics of Continuously Variable Transmissions do not result in lower energy consumption for the four case study vehicles.
This article deals with the dynamic properties of individual wheel electric powertrains for fully electric vehicles, characterised by an in-board location of the motor and transmission, connected to the wheel through half-shafts. Such a layout is applicable to vehicles characterised by significant power and torque requirements where the adoption of in-wheel electric powertrains is not feasible because of packaging constraints. However, the dynamic performance of in-board electric powertrains, especially if adopted for anti-lock braking or traction control, can be affected by the torsional dynamics of the half-shafts. This article presents the dynamic analysis of in-board electric powertrains in both the time domain and the frequency domain. A feedback control system, incorporating state estimation through an extended Kalman filter, is implemented in order to compensate for the effect of the half-shaft dynamics. The effectiveness of the new controller is demonstrated through analysis of the improvement in the performance of the traction control system.
Torque-fill capability during gearshifts is an important customer requirement in automated transmission systems. This functionality can be achieved through transmission system layouts (e.g., based on dual-clutch technology) characterized by significant mechanical complexity, and hence with relatively high cost and mass. This paper describes a parallel hybrid electric drivetrain concept, based on the integration of an electric motor drive into a relatively simple six-speed automated manual transmission. The resulting hybrid electric drivetrain actuates the torque-fill function through control of the electric motor torque during the gearshifts on the engine side of the drivetrain. An optimal controller, based on the off-line computation of the control gain profiles, is presented for the clutch re-engagement phase. The novel controller allows computationally efficient consideration of clutch energy dissipation during the clutch re-engagement phase of the gearshift. The performance with the optimal controller is contrasted with that of two conventional clutch engagement controllers, along a set of gearshifts simulated with an experimentally validated vehicle model.
A system of photovoltaic (PV)-supercapacitor battery is outlined in this study for the charging of a battery for a mid-power electric vehicle (EV) or hybrid vehicle, in conjunction with charging contributions from the grid. Computational models have been developed for each component of the system, namely the PV, supercapacitor, and battery. Model predictions are presented for each system component and validated with experimental data. The required PV area for the charging of the battery of a mid-range EV or hybrid vehicle has also been estimated. © 2014 WIT Press.
This study addresses the development and Hardware-in-the-Loop (HiL) testing of an explicit nonlinear model predictive controller (eNMPC) for an anti-lock braking system (ABS) for passenger cars, actuated through an electro-hydraulic braking (EHB) unit. The control structure includes a compensation strategy to guard against performance degradation due to actuation dead times, identified through experimental tests. The eNMPC is run on an automotive rapid control prototyping unit, which shows its real-time capability with comfortable margin. A validated high-fidelity vehicle simulation model is used for the assessment of the ABS on a HiL rig equipped with the braking system hardware. The eNMPC is tested in 7 emergency braking scenarios, and its performance is benchmarked against a proportional integral derivative (PID) controller. The eNMPC results show: i) the control system robustness with respect to variations of tire-road friction condition and initial vehicle speed; and ii) a consistent and significant improvement of the stopping distance and wheel slip reference tracking, with respect to the vehicle with the PID ABS.
Nonlinear model predictive control (NMPC) is proposed in multiple academic studies as an advanced control system technology for vehicle operation at the limits of handling, allowing high tracking performance and formal consideration of system constraints. However, the implementation of implicit NMPC, in which the control problem is solved on-line, poses significant challenges in terms of computational load. This issue can be overcome through explicit NMPC, in which the optimization problem is solved off-line, and the resulting explicit solution, with guaranteed level of sub-optimality, is evaluated on-line. This study presents a yaw and lateral stability controller based on explicit NMPC, actuated through the friction brakes of the vehicle. The controller performance is demonstrated during sine-with-dwell tests simulated with a high-fidelity model. The analysis investigates the influence of the weights in the cost function formulation and includes a comparison of different settings of the optimal control problem.
The research presented in this paper focuses on the effects of downsizing the electric motor drive of a fully electric vehicle through the adoption of a multiple-speed transmission system. The activity is based on the implementation of a simulation framework in Matlab / Simulink. The paper considers a rear wheel drive case study vehicle, with a baseline drivetrain configuration consisting of a single-speed transmission, which is compared with drivetrains adopting motors with identical peak power but higher base speeds and lower peak torques coupled with multiple-speed transmissions (double and three-speed), to analyze the benefits in terms of energy efficiency and performance. The gear ratios and gearshift maps for each multiple-speed case study are optimized through a procedure developed by the authors consisting of cost functions considering energy efficiency and performance evaluation. The cost functions are explained in the paper along with the models adopted for the research. Copyright © 2012 SAE International.
Future vehicle localisation technologies enable major enhancements of vehicle dynamics control. This study proposes a novel vehicle stability control paradigm, based on pre-emptive control that considers the curvature profile of the expected path ahead in the computation of the reference direct yaw moment and braking control action. The additional information allows pre-emptive trail braking control, which slows down the vehicle if the predicted speed profile based on the current torque demand is deemed incompatible with the reference trajectory ahead. Nonlinear model predictive control is used to implement the approach, in which also the steering angle and reference yaw rate provided to the internal model are varied along the prediction horizon, to account for the expected vehicle path. Two pre-emptive stability control configurations with different levels of complexity are proposed and compared with the passive vehicle, and two state-of-the-art nonlinear model predictive stability controllers, one with and one without non-pre-emptive trail braking control. The performance is assessed along obstacle avoidance tests, simulated with a high-fidelity model of an electric vehicle with in-wheel motors. Results show that the pre-emptive controllers achieve higher maximum entry speeds - up to ∼34% and ∼60% in high and low tyre-road friction conditions - than the formulations without preview.
This paper investigates the potential benefits of photovoltaic (PV) panels on electric vehicles. In addition to the PV panels on the roof of the car, in this study a PV panel is installed below the windshield to increase energy capture when the car is parked. An electro-mechanical actuator makes the PV panel disappear under the roof when the passengers are in the vehicle. The paper presents the simulation model of the overall PV architecture, including the DC/DC converter and the energy storage system. Based on recorded temperature and solar irradiance profiles, the model calculates the energy input and the corresponding range extension. The resulting values are discussed for a prototype four-wheel-drive urban electric vehicle operating in five European locations.
Vehicle handling in steady-state and transient conditions can be significantly enhanced with the continuous modulation of the driving and braking torques of each wheel via dedicated torque-vectoring controllers. For fully electric vehicles with multiple electric motor drives, the enhancements can be achieved through a control allocation algorithm for the determination of the wheel torque distribution. This article analyzes alternative cost functions developed for the allocation of the wheel torques for a four-wheel-driven fully electric vehicle with individually controlled motors. Results in terms of wheel torque and tire slip distributions among the four wheels, and of input power to the electric drivetrains as functions of lateral acceleration are presented and discussed in detail. The cost functions based on minimizing tire slip allow better control performance than the functions based on energy efficiency for the case-study vehicle.
Over the last decade the simulation of driving cycles through longitudinal vehicle models has become an important stage in the design, analysis and selection of vehicle powertrains. This paper presents an overview of existing software packages, along with the development of a new multipurpose driving cycle simulator implemented in the Matlab/Simulink environment. In order to evaluate the performance of the simulator, a MAN TGL 12.240 multi-usage delivery vehicle was fitted with a CAN-bus data logger and used to create a series of 'real-life' drive cycles. These were inputted into the vehicle model and the simulated fuel mass flow-rate and engine rotational speed were compared to those experimentally obtained. Copyright © 2011 SAE International.
An oscillation control system of a vehicle includes a distribution control module configured to determine: a first front torque request for one or more front electric motors of the vehicle; and a first rear torque request for one or more rear electric motors of the vehicle; a first control module configured to: determine a second front torque request for the front electric motor(s) of the vehicle based on the first front torque request and a front wheel road profile; and control power flow to the front electric motor(s) based on the second front torque request; a second control module configured to: determine a second rear torque request for the rear electric motor(s) of the vehicle based on the first rear torque request and a rear wheel road profile; and control power flow to the rear electric motor(s) based on the second rear torque request.
An extensive literature discusses traction control system designs for electric vehicles. In general, the proposed control structures do not include consideration of the actuation dynamics, which are especially important for vehicles with on-board drivetrains, usually characterized by significant torsional dynamics of the half-shafts. This paper compares the performance of a selection of traction controllers from the literature, with that of PID and ∞ control structures specifically designed for on-board electric drivetrains. The analysis in the frequency domain and the simulation results in the time domain show the significant performance improvement provided by the control system designs considering the actuation dynamics.
In car–trailer combinations, the hitch angle is the relative yaw angle between towing car and trailer. The literature has shown that the inclusion of the hitch angle measurement for the feedback control of trailer oscillations can bring safety benefits, compared with conventional trailer sway mitigation algorithms based on the yaw rate of the car. Given the nonlinearity of the vehicle system in the typical conditions requiring the hitch angle control function intervention, nonlinear model-based controllers could be an effective solution. This paper presents four real-time implementable nonlinear model predictive control (NMPC) formulations, using the hitch angle measurement for the torque-vectoring (TV) control of an electric frontwheel drive car towing a trailer. The simulation results show that: (i) the active safety is enhanced by the proposed NMPC TV formulations, with respect to a benchmarking NMPC TV controller only based on the control of the towing car; (ii) the NMPC formulations that directly constrain the hitch angle error, or perform continuous hitch angle tracking, outperform those that modify the reference yaw rate or yaw rate error based on the hitch angle error; and (iii) the NMPC approaches including a dynamic model of the trailer are robust with respect to variations of trailer parameters.
The paper discusses novel computationally efficient torque distribution strategies for electric vehicles with individually controlled drivetrains, aimed at minimizing the overall power losses while providing the required level of wheel torque and yaw moment. Analytical solutions of the torque control allocation problem are derived and effects of load transfers due to moderate driving/braking and cornering conditions are studied and discussed in detail. Influences of different drivetrain characteristics on the front and rear axles are described. The results of the analytically-derived algorithm are contrasted with those from two other control allocation strategies, based on the off-line numerical solution of more detailed formulations of the control allocation problem (i.e., a multi-parametric non-linear programming problem). The solutions of the control allocation problem are experimentally validated along multiple driving cycles and in steady-state cornering, on an electric vehicle with four identical drivetrains. The experiments show that the computationally efficient algorithms represent a very good compromise between low energy consumption and controller complexity.
The paper presents linear and non-linear driveline models for Heavy Goods Vehicles (HGVs) in order to evaluate the main parameters for optimal tuning, when considering the drivability. The implemented models consider the linear and non-linear driveline dynamics, including the effect of the engine inertia, the clutch damper, the driveshaft, the half-shafts and the tires. Sensitivity analyses are carried out for each driveline component during tip-in maneuvers. The paper also analyses the overall frequency response using Bode diagrams and natural frequencies.
This study presents a nonlinear model predictive control (NMPC) formulation for preview-based traction control, which uses the information on the expected tire-road friction coefficient ahead to enhance the wheel slip control performance, in the context of connected vehicles with V2X features. Proof-of-concept experiments on an electric vehicle prototype highlight the real-time capability of the controller, and the wheel slip control performance improvement brought by the tire-road friction coefficient preview. Finally, an experimentally validated simulation model is used in sensitivity analyses, to evaluate the performance benefit of the preview-based controller for different dynamic characteristics (e.g., time constant and pure time delays) of the electric powertrains.
Road irregularities affect vehicle comfort by causing vertical and longitudinal acceleration oscillations. While the current ride comfort enhancement solutions are based on the compensation of the vertical acceleration of the sprung mass, the compensation of the longitudinal dynamics excited by road irregularities has been successfully explored only for in-wheel powertrains. The scope of this study is to demonstrate that also on-board electric powertrains with torsional dynamics of the half-shafts have the potential for effective compensation, thanks to the road profile preview. This paper presents a proof-of-concept nonlinear model predictive controller (NMPC) with road preview, which is assessed with a validated simulation model of an all-wheel drive electric vehicle. Three powertrain layouts are considered, with four in-wheel, four on-board, and two on-board electric machines. The control function is evaluated along multiple manoeuvres, through comfort-related key performance indicators (KPIs) that, for the four on-board layout along a road step test at 40 km/h, highlight >80% improvements. Finally, the real-time implementability of the algorithms is demonstrated, and preliminary experiments are conducted on an electric quadricycle prototype, with more than halved oscillations of the relevant variables.
Catalytic converters mitigate carbon monoxide, hydrocarbon, nitrogen oxides and particulate matter emissions from internal combustion engines, and allow meeting the increasingly stringent emission regulations. However, catalytic converters experience light-off issues during cold start and warm up. This paper reviews the literature on the thermal management of catalysts, which aims to significantly reduce the light-off time and emission concentrations through appropriate heating methods. In particular, methods based on the control of engine parameters are easily implementable, as they do not require extra heating devices. They present good performance in terms of catalyst light-off time reduction, but bring high fuel penalties, caused by the heat loss and unburnt fuel. Other thermal management methods, such as those based on burners, reformers and electrically heated catalysts, involve the installation of additional devices, but allow flexibility in the location and intensity of the heat injection, which can effectively reduce the heat loss in the tailpipe. Heat storage materials decrease catalyst light-off time, emission concentrations and fuel consumption, but they are not effective if the engine remains switched off for long periods of time. The main recommendation of this survey is that integrated and more advanced thermal management control strategies should be developed to reduce light-off time without significant energy penalty.
The design of suspension systems for heavy-duty vehicles covers a specific field of automotive industry. The proposed work focuses on the design development of a front controllable suspension for an agricultural tractor capable to satisfy the system requirements under different operating conditions. The design of the control algorithms is based on the developed multibody model of the actual tractor, including the pitch motion of the sprung mass, the anti-dive effects during braking and forward-reverse maneuvers and the non-linear dynamics of the actuation system. For an advanced analysis, a novel thermo-hydraulic model of the hydraulic system has been implemented. Several semi-active damping controls are analyzed for the specific case study. Therefore, the most promising damping strategy is integrated with other control functions, namely a self-leveling control, an original control algorithm for the reduction of the pitch motion, an anti-impact system for the hydraulic actuator and an on-line adaptation scheme, which preserves an optimal damping ratio of the suspension, even against large variations in operating conditions.
This study investigates the influence of the buried magnet arrangement on the efficiency and drivability performance provided by an on-board interior permanent magnet synchronous machine for a four-wheel-drive electric car with two single-speed on-board powertrains. The relevant motor characteristics, including flux-linkage, inductance, electromagnetic torque, iron loss, total loss, and efficiency, are analyzed for a set of six permanent magnet configurations suitable for the specific machine, which is controlled through maximum-torque-per-ampere and maximum-torque-per-voltage strategies. Moreover, the impact of each magnet arrangement is analyzed in connection with the energy consumption along four driving cycles, as well as the longitudinal acceleration and gradeability performance of the considered vehicle. The simulation results identify the most promising rotor solutions, and show that: (i) the appropriate selection of the rotor configuration is especially important for the driving cycles with substantial high-speed sections; (ii) the magnet arrangement has a major impact on the maximum motor torque below the base speed, and thus on the longitudinal acceleration and gradeability performance; and (iii) the configurations that excel in energy efficiency are among the worst in terms of drivability, and vice versa, i.e., at the vehicle level, the rotor arrangement selection is a trade-off between energy efficiency and longitudinal vehicle dynamics.
In contrast with conventional vehicles driven by an internal-combustion engine, the number of motors in fully electric cars is not fixed. A variety of architectural solutions, including from one to four individually controlled electric drive units, is possible and opens up new avenues in the design of vehicle characteristics. In particular, individual control of multiple electric powertrains promises to enhance the handling performance in steady-state and dynamic conditions. For the analysis and selection of the best electric powertrain layout based on the expected vehicle characteristics and performance, new analytical tools and metrics are required. This article presents and demonstrates a novel offline procedure for the design of the feedforward control action of the vehicle dynamics controller of a fully electric vehicle and three performance indicators for the objective comparison of the handling potential of alternative electric powertrain layouts. The results demonstrate that the proposed offline routine allows the desired understeer characteristics to be achieved with any of the investigated vehicle configurations, in traction and braking conditions. With respect to linear handling characteristics, the simulations indicate that the influence of torque-vectoring is independent of the location of the controlled axles (front or rear) and is considerably affected by the number of controlled axles.
This paper presents a novel four-wheel-drive electric vehicle layout consisting of one on-board electric drivetrain per axle. Each drivetrain includes a simplified clutch-less two-speed transmission system and an open differential to transmit the torque to the wheels. This drivetrain layout allows eight different gear state combinations at the vehicle level, thus increasing the possibility of running the vehicle in a more energy-efficient state for the specific wheel torque demand and speed. To compensate for the torque gap during gearshifts, a "torque-fill" controller was developed that varies the motor torque on the axle not involved in the gearshift. Experimental tests show the effectiveness of the developed gearshift strategy extended with the torque-fill capability. Energy efficiency benefits are discussed by comparing the energy consumptions of the case study vehicle controlled through a constant front-to-total wheel torque distribution and conventional gearshift maps, and the same vehicle with an energy management system based on an offline optimization. Results demonstrate that the more advanced controller brings a significant reduction of the energy consumption at constant speed and along different driving cycles.
The main question in eco-driving is – what speed or torque profile should the vehicle follow to minimize its energy consumption over a certain distance within a desired trip time? Various techniques to obtain globally optimal energy-efficient driving profiles have been proposed in the literature, involving optimization algorithms such as dynamic programming (DP) or sequential quadratic programming. However, these methods are difficult to implement on real vehicles due to their significant computational requirements and the need for precise a-priori knowledge of the scenario ahead. Although many predictions state that electric vehicles (EVs) represent the future of mobility, the literature lacks a realistic analysis of optimal driving profiles for EVs. This paper attempts to address the gap by providing optimal solutions obtained from DP for a variety of trip times, which are compared with simple intuitive speed profiles. For a case study EV, the results show that the DP solutions involve forms of Pulse-and-Glide (PnG) at high frequency. Hence, detailed investigations are performed to: i) prove the optimality conditions of PnG for EVs; ii) show its practical use, based on realistic electric powertrain efficiency maps; iii) propose rules for lower frequency PnG operation; and iv) use PnG to track generic speed profiles.
Electric vehicles with multiple motors allow torque-vectoring, i.e., the individual control of each powertrain torque. Torque-vectoring (TV) can provide: (i) enhancement of vehicle safety and handling, via the generation of a direct yaw moment to shape the understeer characteristics and increase yaw and sideslip damping; and (ii) energy consumption reductions, via appropriate torque allocation to each motor. The FP7 European project iCOMPOSE thoroughly addressed (i) and (ii). Theoretical analyses were carried out to design state-of-the-art TV controllers, which were validated through: (a) vehicle simulations; and (b) extensive experimental tests, which were performed at rolling road facilities and proving grounds, using a Range Rover Evoque prototype equipped with four identical on-board electric powertrains. This paper provides an overview of the TV-related contributions of iCOMPOSE.
The Enhanced Model Reference Adaptive Control (EMRAC) algorithm, augmenting the MRAC strategy with adaptive integral and adaptive switching control actions, is an effective solution to impose reference dynamics to plants affected by parameter uncertainties, unmodeled dynamics and disturbances. However, the design of the EMRAC solutions has so far been limited to single-input systems. To cover the gap, this paper presents two extensions of EMRAC to multi-input systems. The adaptive mechanism of both solutions includes the -modification strategy to assure the boundedness of the adaptive gains also in presence of persistent disturbances. The closed-loop system is analytically studied, and conditions for the asymptotic convergence of the tracking error are presented. Furthermore, when the plant is subjected to unmatched disturbances, the ultimate boundedness of the closed-loop dynamics, which are made discontinuous by the adaptive switching control actions, is systematically proven by using Lyapunov theory for Filippov systems. The problem of trajectory tracking for space robotic arms in presence of unknown and noncooperative targets is used to test the effectiveness of the novel multi-input EMRAC algorithms for taming uncertain systems. Four EMRAC solutions are designed for this engineering application, and tested within a high fidelity simulation framework based on the Robot Operating System. Finally, the tracking performance of the EMRAC implementations is quantitatively evaluated via a set of key performance indicators in the joint space and operational space, and compared with that of four benchmarking controllers.
Anti-jerk controllers actively suppress the torsional oscillations of automotive drivetrains, caused by abrupt variations of the traction torque. The main benefits are: i) enhanced passengers’ comfort; and ii) increased component life. Extensive literature deals with the design of anti-jerk controllers for electric powertrains with on-boardmotors, i.e., in which the electricmotor is part of the sprung mass of the vehicle, and transmits torque to the wheels through a transmission, half-shafts and constant velocity joints. Nevertheless, a complete and structured comparison of the performance of the different control options is still missing. This study addresses the gap through the assessment of six anti-jerk controllers – five exemplary formulations from the literature, and one novel formulation based on explicit nonlinearmodel predictive control (eNMPC). All proposed control structures have the potential to be implemented on production vehicles. A set of objective performance indicators is defined to assess the controllers, which are tuned through an optimization-based routine.Results showthat the wheel speed input is critical to enhance controller performance, but may lead to reduced robustness.
Accelerated Pavement Testing (APT) facilities are nowadays considered fundamental for the thorough understanding of the performance of pavements. The amount of information that can be derived from APT investigations can serve as the basis for a more performance-related pavement design but also for the development of new pavement types and innovative materials. In the design and use of such facilities, special care should be placed in the modeling of the loading systems which are employed to produce accelerated damage. Such an analysis is important both in the preliminary design phase, in which technological solutions are found in order to simulate the effects of heavy vehicles on the pavement, and in the various phases of investigation, when the recorded damage has to be clearly related with effective loading conditions. In this respect, modeling can be a valuable support to the evaluation of the data which can be acquired by means of a proper instrumentation of the facility. In order to address such issues, the Authors have developed a design procedure which together with typical stationary calculations includes the adoption of Multi-Body (MB) and Finite Elements (FE) models of the testing system. As proven by the first implementation exercises of the design procedure, the use of MB and FE models allows the evaluation of the dynamics of the system in a wide variety of testing conditions. Thus, stresses and strains in the structure of the APT facility can be estimated and the dynamic forces and torques which arise during testing at the tire-pavement interface can be predicted. Given the width of the design problem, this paper gives only a general overview of the structure of the proposed procedure, with its application to a specific case which has been studied in depth. Refinements are still under way and will hopefully yield a set of modeling methods which in the future will be available for the design of new APT facilities and for the assessment of the performance of existing systems.
The safety benefits of torque-vectoring control of electric vehicles with multiple drivetrains are well known and extensively discussed in the literature. Also, several authors analyze wheel torque control allocation algorithms for reducing the energy consumption while obtaining the wheel torque demand and reference yaw moment specified by the higher layer of a torque-vectoring controller. Based on a set of novel experimental results, this study demonstrates that further significant energy consumption reductions can be achieved through the appropriate tuning of the reference understeer characteristics. The effects of drivetrain power losses and tire slip power losses are discussed for the case of identical drivetrains at the four vehicle corners. Easily implementable yet effective rule-based algorithms are presented for the set-up of the energy-efficient reference yaw rate, feedforward yaw moment and wheel torque distribution of the torque-vectoring controller.
Next-generation accurate vehicle localization and connectivity technologies will enable significant improvements in vehicle dynamics control. This study proposes a novel control function, referred to as pre-emptive braking, which imposes a braking action if the current vehicle speed is deemed safety-critical with respect to the curvature of the expected path ahead. Differently from the implementations in the literature, the pre-emptive braking input is designed to: a) enhance the safety of the transient vehicle response without compromising the capability of reaching the cornering limit, which is a significant limitation of the algorithms proposed so far; and b) allow - in its most advanced implementation - to precisely constrain the sideslip angle to set levels only through the pre-emptive control of the longitudinal vehicle dynamics, without the application of any direct yaw moment, typical of conventional stability control systems. To this purpose, a real-time-capable nonlinear model predictive control (NMPC) formulation based on a double track vehicle prediction model is presented, and implemented in its implicit form, which is applicable to both human-driven and automated vehicles, and acts as an additional safety function to compensate for human or virtual driver errors in extreme conditions. Its performance is compared with that of: i) two simpler - yet innovative with respect to the state-of-the-art - pre-emptive braking controllers, namely an NMPC implementation based on a dynamic point mass vehicle model, and a pre-emptive rule-based controller; and ii) a benchmarking non-pre-emptive rule-based trail braking controller. The benefits of pre-emptive braking are evaluated through vehicle dynamics simulations with an experimentally validated vehicle model, as well as a proof-of-concept implementation on an automated electric vehicle prototype.
In the last three decades a relatively wide literature has discussed the potential vehicle dynamics benefits of the control of the front-to-total anti-roll moment distribution generated by active suspension systems, either based on actuators located within the individual corners or controllable anti-roll bars. However, because of the nonlinearity of the involved phenomena, there is a lack of systematic model based design routines to achieve the reference cornering response in steady-state and transient conditions through active suspension controllers, and for the integration of suspension control with direct yaw moment control. This paper targets such knowledge gap, by introducing design tools for front-to-total anti-roll moment distribution control, based on: i) optimizations using a quasistatic model for the computation of the non-linear feedforward contribution of the controller; ii) a novel linearized vehicle model formulation for linear control design in the frequency domain; and iii) a nonlinear vehicle model formulation to be used as prediction model for nonlinear model predictive control. A set of simulation and experimental results shows the benefits in terms of: a) understeer gradient tunability; b) increased maximum achievable lateral acceleration; c) increased yaw and sideslip damping; and d) energy consumption reduction.
Fully electric vehicles and range-extended electric vehicles can be characterised by a multitude of possible powertrain layouts, many of them currently under investigation and comparison. This contribution presents a novel clutchless seamless four-speed transmission system which can be concurrently driven by two electric motor drives, for use in fully electric vehicles or electric axles for through-the-road parallel hybrid electric vehicles. The transmission system allows the electric motors to work in their high efficiency region for a longer period during a typical driving schedule. This paper describes the layout of the novel transmission system, the equations for modelling its dynamics and the criteria for the selection of the best gearshift maps for energy efficiency. Finally, an energy consumption and performance comparison between the novel drivetrain, a conventional single-speed electric drivetrain and a double-speed electric drivetrain is discussed in detail for two case study vehicles.
This study presents a traction control system for electric vehicles with in-wheel motors, based on explicit non-linear model predictive control. The feedback law, available beforehand, is described in detail, together with its variation for different plant conditions. The explicit controller is implemented on a rapid control prototyping unit, which proves the real-time capability of the strategy, with computing times in the order of microseconds. These are significantly lower than the required sampling time for a traction control application. Hence, the explicit model predictive controller can run at the same frequency as a simple traction control system based on Proportional Integral (PI) technology. High-fidelity model simulations provide: i) a performance comparison of the proposed explicit non-linear model predictive controller with a benchmark PI-based traction controller with gain scheduling and anti-windup features; and ii) a performance comparison among two explicit and one implicit non-linear model predictive controllers based on different internal models, with and without consideration of transient tire behavior and load transfers. Experimental test results on an electric vehicle demonstrator are shown for one of the explicit non-linear model predictive controller formulations.
A significant challenge in electric vehicles with multiple motors is how to control the individual drivetrains in order to achieve measurable benefits in terms of vehicle cornering response, compared to conventional stability control systems actuating the friction brakes. This paper presents a direct yaw moment controller based on the combination of feedforward and feedback contributions for continuous yaw rate control. When the estimated sideslip exceeds a pre-defined threshold, a sideslip-based yaw moment contribution is activated. All yaw moment contributions are entirely tunable through model-based approaches, for reduced vehicle testing time. The purpose of the controller is to continuously modify the vehicle understeer characteristic in quasi-static conditions and increase yaw and sideslip damping during transients. Skid-pad, step-steer and sweep steer tests are carried out with a front-wheel-drive fully electric vehicle demonstrator with two independent drivetrains. The experimental test results of the electric motor-based actuation of the direct yaw moment controller are compared with those deriving from the friction brake-based actuation of the same algorithm, which is a major contribution of this paper. The novel results show that continuous direct yaw moment control allows significant "on-demand" changes of the vehicle response in cornering conditions and to enhance active vehicle safety during extreme driving maneuvers.
State-of-the-art antilock braking systems (ABS) are reactive, i.e., they activate after detecting that wheels tend to lock in braking. With vehicle-to-everything (V2X) connectivity becoming a reality, it will be possible to gather information on the tire-road friction conditions ahead, and use these data to enhance wheel slip control performance, especially during abrupt friction level variations. This study presents a nonlinear model predictive controller (NMPC) for ABS with preview of the tire-road friction profile. The potential benefits, optimal prediction horizon, and robustness of the preview algorithm are evaluated for different dynamic characteristics of the brake actuation system, through an experimentally validated simulation model. Proof-of-concept experiments with an electric vehicle prototype highlight the real-time capability of the proposed NMPC ABS, and the associated wheel slip control performance improvements in braking maneuvers with high-to-low friction transitions.
With the advent of electric vehicles with multiple motors, the steady-state and transient cornering responses can be designed based on high-level reference targets, and implemented through the continuous torque control of the individual wheels, i.e., torque-vectoring or direct yaw moment control. The literature includes several papers describing the application of the sliding mode control theory to torque-vectoring. However, the experimental implementations of sliding mode controllers on real vehicle prototypes are very limited at the moment. More importantly, to the knowledge of the authors, there is lack of experimental assessments of the performance benefits of direct yaw moment control based on sliding modes, with respect to other controllers, such as the proportional integral derivative controllers or linear quadratic regulators currently used for stability control in production vehicles. This paper aims to reduce this gap by presenting an integral sliding mode controller for concurrent yaw rate and sideslip control. A new driving mode, the Enhanced Sport mode, is proposed, inducing sustained high values of sideslip angle, which can be safely limited to a specified threshold. The system is experimentally assessed on a four-wheel-drive electric vehicle along a wide range of maneuvers. The performance of the integral sliding mode controller is compared with that of a linear quadratic regulator during step steer tests. The results show that the integral sliding mode controller brings a significant enhancement of the tracking performance and yaw damping with respect to the more conventional linear quadratic regulator based on an augmented single-track vehicle model formulation.
Path tracking (PT) controllers capable of replicating race driving techniques, such as drifting beyond the limits of handling, have the potential of enhancing active safety in critical conditions. This paper presents a nonlinear model predictive control (NMPC) approach that integrates multiple actuation methods, namely four-wheel-steering, longitudinal tyre force distribution, and direct yaw moment control, to execute drifting when this is beneficial for PT in emergency scenarios. Simulation results of challenging manoeuvres, based on an experimentally validated vehicle model, highlight the substantial PT performance improvements brought by: i) vehicle operation outside the envelope enforced by the current generation of stability controllers; and ii) the integrated control of multiple actuators.
Electric vehicles (EVs) are characterized by a significant variety of possible powertrain configurations, ranging from one to four electric machines, which can have an on-board or in-wheel layout. Multiple models of production EVs have recently been introduced on the market, with 4-wheel-drive (4WD) architectures based on a central motor within each axle, connected to the wheels through a gearbox, a differential, and half-shafts. In parallel, an important body of research and industrial demonstrations have covered the topic of 2-speed transmission systems for EVs, with the target of enhancing longitudinal acceleration and gradeability performance, while increasing the operating efficiency of the electric powertrain. Although several recent studies compare different electric powertrain architectures, to the best of the authors’ knowledge the literature misses a comparison between 2-wheel-drive (2WD) and 4WD configurations for the same EV, from the viewpoint of drivability and energy consumption. This paper targets this gap, by assessing 2WD and 4WD powertrain layouts with central motors, for a case study light passenger car for urban mobility, including consideration of the effect of single- and 2-speed transmission systems. An optimization routine is used to calculate the energy-efficient gear state and/or torque distribution for each considered configuration. For the specific EV, the results highlight the favourable trade-off of the single-speed 4WD layout, capable of reducing the energy consumption during driving cycles by approximately 9% with respect to the conventional 2WD layout with single-speed transmission, while providing satisfactory drivability and good gradeability, especially in low tire–road friction conditions.
Electric vehicles with independently controlled drivetrains allow torque-vectoring, which enhances active safety and handling qualities. This paper proposes an approach for the concurrent control of yaw rate and sideslip angle based on a single input single output (SISO) yaw rate controller. With the SISO formulation, the reference yaw rate is firstly defined according to the vehicle handling requirements, and is then corrected based on the actual sideslip angle. The sideslip angle contribution guarantees a prompt corrective action in critical situations such as incipient vehicle oversteer during limit cornering in low tire-road friction conditions. A design methodology in the frequency domain is discussed, including stability analysis based on the theory of switched linear systems. The performance of the control structure is assessed via: i) phase-plane plots obtained with a non-linear vehicle model; ii) simulations with an experimentally validated model, including multiple feedback control structures; and iii) experimental tests on an electric vehicle demonstrator along step steer maneuvers with purposely induced and controlled vehicle drift. Results show that the SISO controller allows constraining the sideslip angle within the predetermined thresholds and yields tire-road friction adaptation with all the considered feedback controllers.
Smart tires are systems that are able to measure temperature, inflation pressure, footprint dimensions, and, importantly, tire contact forces. The integration of this additional information with the signals ob-tained from more conventional vehicle sensors, e.g., inertial measure-ment units, can enhance state estimation in production cars. This paper evaluates the use of smart tires to improve the estimation performance of an Unscented Kalman filter (UKF) based on a nonlinear vehicle dynam-ics model. Two UKF implementations, excluding and including smart tire information, are compared in terms of estimation accuracy of vehicle speed, sideslip angle and tire-road friction coefficient, using experi-mental data obtained on a high performance passenger car.
The EVC1000 project (Electric Vehicle Components for 1000 km daily trips) aims at developing brand-independent components and systems, and demonstrates them through an integrated wheel-centric propulsion architecture and EV (Electric Vehicle) management approach implemented on two different EVs. The project relies on in-wheel motor and provides new chassis components and integrated controllers. Moreover a compact centralised drive for in-wheel motor axles, based on Silicon Carbide technology, targeting superior levels of functional integration and failsafe operation will be integrated in the EVs.
V2X connectivity and powertrain electrification are emerging trends in the automotive sector, which enable the implementation of new control solutions. Most of the production electric vehicles have centralized powertrain architectures consisting of a single central on-board motor, a single-speed transmission, an open differential, half-shafts, and constant velocity joints. The torsional drivetrain dynamics and wheel dynamics are influenced by the open differential, especially in split- scenarios, i.e., with different tire-road friction coefficients on the two wheels of the same axle, and are attenuated by the so-called anti-jerk controllers. Although a rather extensive literature discusses traction control formulations for individual wheel slip control, there is a knowledge gap on: a) model based traction controllers for centralized powertrains; and b) traction controllers using the preview of the expected tire-road friction condition ahead, e.g., obtained through V2X, for enhancing the wheel slip tracking performance. This study presents nonlinear model predictive control formulations for traction control and anti-jerk control in electric powertrains with central motor and open differential, and benefitting from the preview of the tire-road friction level. The simulation results in straight line and cornering conditions, obtained with an experimentally validated vehicle model, as well as the proof-of-concept experiments on an electric quadricycle prototype, highlight the benefits of the novel controllers.
In energy storage devices carbonaceous composite electrodes are a popular choice, consisting of activated carbon (ac), conductive additives and a polymeric binder matrix. The active electrode components are in the form of ac particles, ac fibres, or ac monolith combined with conductive additives such as carbon black. Activated carbon plays the most important role for storing a large amount of energy in the form of ions contained in the carbon nanopores. This study considers a modelling approach to the meso-nano and micro-nano infiltration of ions into the porous carbon structure during the operation of the energy storage device. Depending on the pore size, ion size and solvent molecule size, ions may be solvated or unsolvated as they move, where ions are solvated in meso-pores for most cases. Molecular model simulations have been performed to determine the values of the geometrical parameters of different ions, solvated and unsolvated in various solvents. A meso-nano and micro-nano ion infiltration model has been developed in this study under both steady state and dynamic conditions.
The combination of continuously-acting high level controllers and control allocation techniques allows various driving modes to be made available to the driver. The driving modes modify the fundamental vehicle performance characteristics including the understeer characteristic and also enable varying emphasis to be placed on aspects such as tire slip and energy efficiency. In this study, control and wheel torque allocation techniques are used to produce three driving modes. Using simulation of an empirically validated model that incorporates the dynamics of the electric powertrains, the vehicle performance, longitudinal slip and power utilization during straight-ahead driving and cornering maneuvers under the different driving modes are compared. The three driving modes enable significant changes to the vehicle behavior to be induced, allowing the responsiveness of the car to the steering wheel inputs and the lateral acceleration limits to be varied according to the selected driving mode. Furthermore, the different driving modes have a significant impact on the longitudinal tire slip, the motor power losses and the total power utilization. The control and wheel torque allocation methods do not rely on complex and computationally demanding online optimization schemes and can thus be practically implemented on real fully electric vehicles. Copyright © 2014 SAE International.
Anti-jerk controllers compensate for the torsional oscillations of automotive drivetrains, caused by swift variations of the traction torque. In the literature model predictive control (MPC) technology has been applied to anti-jerk control problems, by using a variety of prediction models. However, an analysis of the influence of the prediction model complexity on anti-jerk control performance is still missing. To cover the gap, this study proposes six anti-jerk MPC formulations, which are based on different prediction models and are fine-tuned through a unified optimization routine. Their performance is assessed over multiple tip-in and tip-out maneuvers by means of an objective indicator. Results show that: i) low number of prediction steps and short discretization time provide the best performance in the considered nominal tip-in test; ii) the consideration of the drivetrain backlash in the prediction model is beneficial in all test cases; iii) the inclusion of tire slip formulations makes the system more robust with respect to vehicle speed variations and enhances the vehicle behavior in tip-out tests; however, it deteriorates performance in the other scenarios; and iv) the inclusion of a simplified tire relaxation formulation does not bring any particular benefit.
Anti-jerk controllers, commonly implemented in production vehicles, reduce the longitudinal acceleration oscillations transmitted to the passengers, which are caused by the torsional dynamics of the drivetrain during torque transients. Hence, these controllers enhance comfort, drivability, and drivetrain compo- nent durability. Although anti-jerk controllers are commonly implemented in conventional production internal-combustion-engine-driven vehicles, the topic of anti-jerk control has recently been the subject of increased academic and industrial interest, because of the trend towards powertrain electrification, and the distinctive features of electric powertrains, such as the high torque generation bandwidth and absence of clutch dampers. This paper reviews the state-of-the-art of automotive anti-jerk control, with particular attention to control structures that are practically implementable on real vehicles. The survey starts with an overview of the causes of the longitudinal vehicle acceleration oscillations that follow abrupt changes in the powertrain torque delivery. The main body of the text reviews examples of anti-jerk controllers, and categorizes them according to the adopted error variable. The ancillary functions of typical anti-jerk controllers, e.g., their activation and deactivation conditions, are explained. The paper concludes with the most recent development trends, and ideas for future work, including possible applications of model pre- dictive control as well as integration of anti-jerk controllers with autonomous driving systems and other vehicle control functions.
Tire forces are at the heart of the dynamic qualities of vehicles. With the advent of electric vehicles the precise and accurate control of the traction and braking forces at the individual wheel becomes a possibility and a reality outside test labs and virtual proving grounds. Benefits of individual wheel torque control, or torque-vectoring, in terms of vehicle dynamics behavior have been well documented in the literature. However, very few studies exist which analyze the individual wheel torque control integrated with vehicle efficiency considerations. This paper focuses on this aspect and discusses the possibilities and benefits of integrated, energy efficient torque vectoring control. Experiments with a four-wheel-drive electric vehicle show that considerable energy savings can be achieved by considering drivetrain and tire power losses through energy efficient torque vectoring control.
The main benefits of dual clutch transmissions (DCTs) are: (i) a higher energy efficiency than automatic transmission systems with torque converters; and (ii) the capability to fill the torque gap during gear shifts to allow seamless longitudinal acceleration profiles. Therefore, DCTs are viable alternatives to automated manual transmissions (AMTs). For vehicles equipped with engines that can generate considerable torque, large clutch-slip energy losses occur during power-on gear shifts and, as a result, DCTs need wet clutches for effective heat dissipation. This requirement substantially reduces DCT efficiency because of the churning and ancillary power dissipations associated with the wet clutch pack. To the knowledge of the authors, this study is the first to analyse the detailed power loss contributions of a DCT with wet clutches, and their relative significance along a set of driving cycles. Based on these results, a novel hybridised AMT (HAMT) with a single dry clutch and an electric motor is proposed for the same vehicle. The HAMT architecture combines the high mechanical efficiency typical of AMTs with a single dry clutch, with the torque-fill capability and operational flexibility allowed by the electric motor. The measured efficiency maps of a case study DCT and HAMT are compared. This is then complemented by the analysis of the respective fuel consumption along the driving cycles, which is simulated with an experimentally validated vehicle model. In its internal combustion engine mode, the HAMT reduces fuel consumption by >9% with respect to the DCT.
E-mobility is a game changer for the automotive domain. It promises significant reduction in terms of complexity and in terms of local emissions. With falling prices and recent technological advances, the second generation of electric vehicles (EVs) that is now in production makes electromobility an affordable and viable option for more and more transport mission (people, freight). Still, major challenges for large scale deployment remain. They include higher maturity with respect to performance (e.g., range, interaction with the grid), development efficiency (e.g., time-to-market), or production costs. Additionally, an important market transformation currently occurs with the co-development of automated driving functions, connectivity, mobility-as-a-service. New opportunities arise to customize road transportation systems toward application-driven, user-centric smart mobility solutions. The target of this paper is to provide a consolidated view of several related European research programs having the common goal to develop innovative, brand-independent architectures, components and systems for next generation electrified vehicles optimised for the infrastructure under the umbrella of the E-VOLVE cluster. This regroups the projects ACHILES, SYS2WHEEL, EVC1000 introducing innovative in-wheel motors for different vehicle segments, CEVOLVER introducing optimized concepts for energy and thermal management, and Multi-Moby focusing on the development of safe, efficient and affordable urban electric vehicles.
Many torque-vectoring controllers are based on the concurrent control of yaw rate and sideslip angle through complex multi-variable control structures. In general, the target is to continuously track a reference yaw rate, and constrain the sideslip angle to remain within thresholds that are critical for vehicle stability. To achieve this objective, this paper presents a single input single output (SISO) formulation, which varies the reference yaw rate to constrain sideslip angle. The performance of the controller is successfully validated through simulations and experimental tests on an electric vehicle prototype with four drivetrains
Electric vehicles with multiple motors allow torque-vectoring, which generates a yaw moment by assigning different motor torques at the left and right wheels. This permits designing the steady-state cornering response according to several vehicle handling quality targets. For example, as widely discussed in the literature, to make the vehicle more sports-oriented, it is possible to reduce the understeer gradient and increase the maximum lateral acceleration with respect to the same vehicle without torque-vectoring. This paper focuses on the novel experimentally-based design of a reference vehicle understeer characteristic providing energy efficiency enhancement over the whole range of achievable lateral accelerations. Experiments show that an appropriate tuning of the reference understeer characteristic, i.e., the reference yaw rate of the torque-vectoring controller, can bring energy savings of up to ~11% for a case study four-wheel-drive electric vehicle demonstrator. Moreover, during constant speed cornering, it is more efficient to significantly reduce the level of vehicle understeer, with respect to the same vehicle with even torque distribution on the left and right wheels.
© 2012 WEVA.This paper deals with the description of current and future vehicle technology related to yaw moment control, anti-lock braking and traction control through the employment of effective torque vectoring strategies for electric vehicles. In particular, the adoption of individually controlled electric powertrains with the aim of tuning the vehicle dynamic characteristics in steady-state and transient conditions is discussed. This subject is currently investigated within the European Union (EU) funded Seventh Framework Programme (FP7) consortium E-VECTOORC, focused on the development and experimental testing of novel control strategies. Through a comprehensive literature review, the article outlines the stateof- the-art of torque vectoring control for fully electric vehicles and presents the philosophy and the potential impact of the E-VECTOORC control structure from the viewpoint of torque vectoring for vehicle dynamics enhancement.
The electric vehicle is becoming increasingly prevalent as a viable option to replace hydrocarbon fuelled vehicles, and as such the development of high efficiency fully electric drivetrains is a particularly relevant research topic. The drivetrain topology is one of the main focuses of research on fully electric drivetrains, because of the variety of available options. For example, the adoption of multiple-speed mechanical transmissions can improve both the performance and energy consumption when compared to a single-speed transmission. A four-speed, dual motor drivetrain design is presented in this article which works on the principle of two double-speed transmissions, each driven by a separate motor linked through a sole secondary shaft. This drivetrain architecture provides increased flexibility of the electric motor operating points, theoretically being beneficial to the overall efficiency of the system for any driving condition. This paper presents the design of the transmission, its governing equations and the method adopted to optimize the state selection map and electric motor torque distribution. A backward-facing energy consumption model is used to compare the results of the four-speed transmission with those of single- and double-speed transmissions for four case study vehicles. © 2012 IEEE.
The handling characteristic is a classical topic of vehicle dynamics. Usually, vehicle handling is studied through the analysis of the understeer coefficient in quasi-steady-state maneuvers. In this paper, experimental tests are performed on an electric vehicle with four independent motors, which is able to reproduce front-wheel-drive, rear-wheel-drive and all-wheel-drive (FWD, RWD and AWD, respectively) architectures. The handling characteristics of each architecture are inferred through classical and new concepts. More specifically, the study presents a procedure to compute the longitudinal and lateral tire forces, which is based on a first estimate and a subsequent correction of the tire forces that guarantee the equilibrium. A yaw moment analysis is then performed to identify the contributions of the longitudinal and lateral forces. The results show a good agreement between the classical and new formulations of the understeer coefficient, and allow to infer a relationship between the understeer coefficient and the yaw moment analysis. The handling characteristics for the considered maneuvers vary with the vehicle speed and front-to-rear wheel torque distribution. In particular, an apparently surprising result arises at low speed, where the RWD architecture is the most understeering configuration. This outcome is discussed through the yaw moment analysis, highlighting the yaw moment caused by the longitudinal forces of the front tires, which is significant for high values of lateral acceleration and steering angle.
The design and optimisation of a permanent magnet-assisted synchronous reluctance (PMaSynR) traction machine is described to improve its energy efficiency over a selection of driving cycles, when installed on a four-wheel-drive electrically powered vehicle for urban use, with two on-board powertrains. The driving cycle-based optimisation is defined with the objective of minimising motor energy loss under strict size constraints, while maintaining the peak torque and restricting the torque ripple. The key design parameters that exert the most significant influence on the selected performance indicators are identified through a parametric sensitivity analysis. The optimisation brings a motor design that is characterised by an energy loss reduction of 8.2% over the WLTP Class 2 driving cycle and 11.7% over the NEDC and Artemis Urban driving cycles, at the price of a 4.7% peak torque reduction with respect to the baseline machine. Additional analysis, implemented outside the optimisation framework, revealed that different coil turn adjustments would reduce the energy loss along the considered driving cycles. However, under realistic size constraints, the optimal design solutions are the same.
This paper discusses the vehicle dynamics control system of an all-wheel-drive electric vehicle with four on-board motors. Each driveline is characterized by complex torsional dynamics caused by the gearbox and the half-shaft located between the electric motor and the wheel. Such a drivetrain configuration can benefit from a specific controller, i.e., the active vibration controller (AVC), which increases the damping ratio of the actuation system. The AVC enhances the effectiveness of the other vehicle dynamics controllers such as the direct yaw moment controller (DYC) and the wheel slip controllers (WSCs). This study introduces examples of AVC, DYC and WSC implementations, and their experimental validation on the electric vehicle demonstrator of the European Union FP7 E-VECTOORC project. The experimental results confirm that the powertrain architecture with individually controlled on-board motors significantly improves the traction, braking and cornering capabilities of the electric vehicle, while increasing comfort and active safety.
The market penetration of electric vehicles (EVs) is going to significantly increase in the next years and decades. However, EVs still present significant practical limitations in terms of mileage. Hence, the automotive industry is making important research efforts towards the progressive increase of battery energy density, reduction of battery charging time, and enhancement of electric powertrain efficiency. The electric machine is the main power loss contributor of an electric powertrain. This literature survey reviews the design and control methods to improve the energy efficiency of electric machines for EVs. The motor design requirements and specifications are described in terms of power density, efficiency along driving cycles, and cost, according to the targets set by the roadmaps of the main governmental agencies. The review discusses the stator and rotor design parameters, winding configurations, novel materials, construction technologies as well as control methods that are most influential on the power loss characteristics of typical traction machines. Moreover, the paper covers: i) driving cycle based design methods of traction motors, for energy consumption reduction in real operating conditions; and ii) novel machine topologies providing potential efficiency benefits.
Steering control for path tracking in autonomous vehicles is well documented in the literature. Also, continuous direct yaw moment control, i.e., torque-vectoring, applied to human-driven electric vehicles with multiple motors is extensively researched. However, the combination of both controllers is not yet well understood. This paper analyzes the benefits of torque-vectoring in an autonomous electric vehicle, either by integrating the torque-vectoring system in the path tracking controller, or through its separate implementation alongside the steering controller for path tracking. A selection of path tracking controllers is compared in obstacle avoidance tests simulated with an experimentally validated vehicle dynamics model. A genetic optimization is used to select the controller parameters. Simulation results confirm that torque-vectoring is beneficial to autonomous vehicle response. The integrated controllers achieve the best performance if they are tuned for the specific tire-road friction condition. However, they can also cause unstable behavior when they operate in lower friction conditions without any re-tuning. On the other hand, separate torque-vectoring implementations provide consistently stable cornering response for a wide range of friction conditions. Controllers with preview formulations, or based on appropriate reference paths with respect to the middle line of the available lane, are beneficial to the path tracking performance.
Individually-controlled powertrains of fully electric vehicles present an opportunity to enhance the steady-state and transient cornering response of a car via continuously-acting controllers and enable various “driving modes” to be available. This study investigates the associated potential for energy savings through the minimization of power losses from the motor units via wheel torque allocation. Power losses in straight-ahead driving and a ramp steer maneuver for different motor types and under different wheel torque allocation schemes are analyzed in an offline simulation approach. Significant reductions in motor power losses are achieved for two motor types using an optimization scheme based on look-up tables of motor loss data. Energy loss minimization cannot be achieved through a direct quadratic approximation of the power losses.
Latest advances in road profile sensors make the implementation of pre-emptive suspension control a viable option for production vehicles. From the control side, model predictive control (MPC) in combination with preview is a powerful solution for this application. However, the significant computational load associated with conventional implicit model predictive controllers (i-MPCs) is one of the limiting factors to the widespread industrial adoption of MPC. As an alternative, this paper proposes an explicit model predictive controller (e-MPC) for an active suspension system with preview. The MPC optimization is run offline, and the online controller is reduced to a function evaluation. To overcome the increased memory requirements, the controller uses the recently developed regionless e-MPC approach. The controller was assessed through simulations and experiments on a sport utility vehicle demonstrator with controllable hydraulic suspension actuators. For frequencies
In the last decade, Hybrid Electric Vehicles (HEVs) have spread worldwide due to their capability to reduce fuel consumption. Several studies focused on the optimisation of the energy management system of hybrid vehicles are available in literature, whilst there are few articles dealing with the drivability and the dynamics of these new powertrain systems. In this paper a 'Through-the-Road-Parallel HEV' is analysed. This architecture is composed of an internal combustion engine mounted on the front axle and an electric motor powering the rear one. These two powertrains are not directly connected to each other, as the parallel configuration is implemented through the road-tyre force interaction. The main purpose of this paper is the drivability analysis of this layout of HEVs, using linearised mathematical models in both time (i.e. vehicle response during tip-in tests) and frequency domain (i.e. frequency response functions), considering the effect of the engaged gear ratio. The differences from a traditional Front-Wheel-Drive (FWD) configuration are subsequently highlighted. Furthermore, the authors compare different linearised dynamic models, with an increasing number of degrees of freedom, in order to assess which model represents the best compromise between complexity and quality of the results. Finally, a sensitivity analysis of the influence of the torque distribution between the front (thermal) and rear (electric) axles on vehicle drivability is carried out and presented in detail. © 2012 Springer Science+Business Media Dordrecht.
In track-oriented road cars with electric powertrains, the ability to recuperate energy during track driving is significantly affected by the frequent interventions of the antilock braking system (ABS), which usually severely limits the regenerative torque level because of functional safety considerations. In high-performance vehicles, when controlling an active rear wing to maximize brake regeneration, it is unclear whether it is preferable to maximize drag by positioning the wing into its stall position, to maximize downforce, or to impose an intermediate aerodynamic setup. To maximize energy recuperation during braking from high speeds, this paper presents a novel integrated open-loop strategy to control: (i) the orientation of an active rear wing; (ii) the front-to-total brake force distribution; and (iii) the blending between regenerative and friction braking. For the case study wing and vehicle setup, the results show that the optimal wing positions for maximum regeneration and maximum deceleration coincide for most of the vehicle operating envelope. In fact, the wing position that maximizes drag by causing stall brings up to 37% increased energy recuperation over a passive wing during a braking maneuver from 300 km/h to 50 km/h by preventing the ABS intervention, despite achieving higher deceleration and a 2% shorter stopping distance. Furthermore, the maximum drag position also reduces the longitudinal tire slip power losses, which, for example, results in a 0.4% recuperated energy increase when braking from 300 km/h to 50 km/h in high tire–road friction conditions at a deceleration close to the limit of the vehicle with passive aerodynamics, i.e., without ABS interventions.
This paper investigates a torque-vectoring formulation for the combined control of the yaw rate and hitch angle of an articulated vehicle through a direct yaw moment generated on the towing car. The formulation is based on a single-input single-output feedback control structure, in which the reference yaw rate for the car is modified when the incipient instability of the trailer is detected with a hitch angle sensor. The design of the hitch angle controller is described, including the gain scheduling as a function of vehicle speed. The controller performance is assessed by means of frequency domain and phase plane analyses, and compared with that of an industrial trailer sway mitigation algorithm. In addition, the novel control strategy is implemented in a high-fidelity articulated vehicle model for robustness assessment, and experimentally tested on an electric vehicle demonstrator with four on-board drivetrains, towing two different conventional single-axle trailers. The results show that: (i) the torque-vectoring controller based only on the yaw rate of the car is not sufficient to mitigate trailer instability in extreme conditions; and (ii) the proposed controller provides safe trailer behaviour during the comprehensive set of manoeuvres, thus justifying the additional hardware complexity associated with the hitch angle measurement.
E-mobility is a game changer for the automotive domain. It promises significant reduction in terms of complexity and in terms of local emissions. With falling prices and recent technological advances, the second generation of electric vehicles (EVs) that is now in production makes electromobility an affordable and viable option for more and more transport mission (people, freight). Current e-vehicle platforms still present architectural similarities with respect to combustion engine vehicle (e.g., centralized motor). Target of the European project EVC1000 is to introduce corner solutions with in-wheel motors supported by electrified chassis components (brake-by-wire, active suspension) and advanced control strategies for full potential exploitation. Especially, it is expected that this solution will provide more architectural freedom toward “design-for-purpose” vehicles built for dedicated usage models, further providing higher performances. Target of this paper are (a) to introduce the EVC1000 project and results achieved so far; (b) with the example of two vehicle platforms (AUDI E-tron and JAC iEV7) to discuss platform migration opportunities and challenges related to corner solutions, and (c) to present preliminary results (simulation based) with respect to expected performance increase.
This paper describes a torque-vectoring (TV) algorithm for the control of the hitch angle of an articulated vehicle. The hitch angle control function prevents trailer oscillations and instability during extreme cornering maneuvers. The proposed control variable is a weighted combination of terms accounting for the yaw rate, sideslip angle and hitch angle of the articulated vehicle. The novel control variable formulation results in a single-input single-output (SISO) feedback controller. In the specific application a simple proportional integral (PI) controller with gain scheduling on vehicle velocity is developed. The TV system is implemented and experimentally tested on a fully electric vehicle with four on-board drivetrains, towing a single-axle passive trailer. Sinusoidal steer test results show that the proposed algorithm significantly improves the behavior of the articulated vehicle, and justify further research on the topic of hitch angle control through TV.
Automotive suspension systems are key to ride comfort and handling performance enhancement. In the last decades semi-active and active suspension configurations have been the focus of intensive automotive engineering research, and have been implemented by the industry. The recent advances in road profile measurement and estimation systems make road-preview-based suspension control a viable solution for production vehicles. Despite the availability of a significant body of papers on the topic, the literature lacks a comprehensive and up-to-date survey on the variety of proposed techniques for suspension control with road preview, and the comparison of their effectiveness. To cover the gap, this literature review deals with the research conducted over the past decades on the topic of semi-active and active suspension controllers with road preview. The main formulations are reported for each control category, and the respective features are critically analysed, together with the most relevant performance indicators. The paper also discusses the effect of the road preview time on the resulting system performance, and identifies control development trends.
Vehicle platooning provides avenues to improve road transportation byreducingenergyconsumptionandpollutantemissionswhileincreasingsafety androadusage.However,tosafelyoperateasequenceofconnectedautonomous vehicles(CAVs)as a platoon, stringent conditions, such as string stability, on the closed-loop platoon dynamics must be guaranteed. To meet such platoon control specifications, linear longitudinal vehicle dynamics are usually assumed for the design of platoon control algorithms, and are imposed to each vehicle in the platoon by using mid-level control systems. However, disturbances and model mismatches can limit the compensation of the nonlinear vehicle dynamics, and thus jeopardise the tracking of the reference linear behaviour. To systematically impose the linear behaviour to the vehicles in a platoon despite model uncertainties and disturbances, in this paper two adaptive solutions are proposed and compared: (i) an adaptive solution for systems in Brunovsky form; and (ii) the Enhanced Model Reference Adaptive Control. Numerical results confirm that both adaptive techniques are effective in imposing the linear dynamics to the longitudinal vehicle motion, also when integrated within a platoon control architecture. The closed-loop platoon performance is assessed via a set of performance indicators for different platoon lengths.
Among other variables, smart tyre systems are capable of determining tyre contact forces. Combining this information with the signals obtained from conventional vehicle sensors, e.g. inertial measurement units, wheel speed sensors and steering wheel angle sensors, improves the estimation accuracy of the states used by the vehicle dynamics controllers of production cars. This study assesses the performance improvement brought by the vertical and longitudinal tyre contact force signals, obtained through smart tyres, to an unscented Kalman filter (UKF) for vehicle speed and sideslip angle estimation, based on a nonlinear vehicle dynamics model. Two UKF designs, excluding and including smart tyre information, are compared by using experimental data from a purposely sensorised high-performance passenger car, along a comprehensive set of manoeuvres. The results show average ∼60% and ∼37% reductions of the root mean square values of the normalised vehicle speed and sideslip angle estimation errors for the filter design receiving the smart tyre information. In comparison with the more conventional UKF design without tyre force inputs, the smart tyre based estimator is also characterised by significantly enhanced robustness and adaptability to typical variations of vehicle and tyre parameters, such as the tyre-road friction coefficient.
Vehicles equipped with Automated Manual Transmissions (AMT) for gear shift control show many advantages in terms of reduction of fuel consumption and improvement of driving comfort and shifting quality. In order to increase both performance and efficiency, an important target is focused on the minimization of the typical torque interruption during the gear shift, especially in front of the conventional automatic transmission. Recently, AMT are proposed to be connected with planetary gears and friction brakes, in order to reduce the torque gap during the gear change process. This paper is focused on a block-oriented simulation methodology developed in Matlab/Simulink/ Stateflow environment, able to simulate the performance of a complete FWD powertrain and in particular to predict dynamic performance and overall efficiency of the AMT with innovative Torque Gap Filler devices (TGF). A comparison between traditional Manual Transmission (MT) system and AMT with TGF device is presented, focusing in particular on the gear shift control strategies able to improve the performance of these systems. Some dynamic simulations are discussed in order to validate the proposed model and to simulate performance and limitations of this mechanical solution. Copyright © 2008 SAE International.
In vehicle dynamics, yaw rate control is used to improve the cornering response in steady-state and transient conditions. This can be achieved through an appropriate anti-roll moment distribution between the front and rear axles of a vehicle with controllable suspension actuators. Such control action alters the load transfer distribution, which in turn provokes a lateral tire force variation. With respect to the extensive set of papers from the literature discussing yaw rate tracking through active suspension control, this study presents: i) A detailed analysis of the effect of the load transfer on the lateral axle force and cornering stiffness; ii) A novel linearized single-track vehicle model formulation for control system design, based on the results in i); and iii) An optimization-based routine for the design of the non-linear feedforward contribution of the control action. The resulting feedforward-feedback controller is assessed through: a) Simulations with an experimentally validated model of a vehicle with active anti-roll bars (case study 1); and b) Experimental tests on a vehicle prototype with an active suspension system (case study 2).
The benefits of individual wheel torque control, or torque vectoring, in terms of vehicle dynamics behaviour have been well documented in the literature. However, few studies analyse individual wheel torque control integrated with electric vehicle efficiency considerations. The possibilities and benefits of energy efficient torque vectoring control for electric vehicles with multiple drivetrains are discussed. In particular, energy consumption reductions can be obtained through specific design of the wheel torque control allocation algorithm and the reference yaw rate characteristics. Experiments with a four-wheel-drive electric vehicle demonstrate considerable energy savings.
Research and development of energy storage systems are almost entirely dominated by the need for continuously increasing their performance, reducing weight, cost, and increasing efficiency. The combination of battery and supercapacitor within the hybrid energy storage system (HESS) involves the management of the power between the two devices. The objective of this study is to develop a novel model predictive controller for the HESS of a through-the- road-parallel hybrid electric vehicle, which aims to extend the battery life. Results in terms of battery life expectancy and HESS energy efficiency are presented and discussed. © 2013 IEEE.
Individually-controlled power trains of fully electric vehicles present an opportunity to enhance the steady-state and transient cornering response of a car via continuously-acting controllers and enable various "driving modes" to be available. This study investigates the associated potential for energy savings through the minimization of power losses from the motor units via wheel torque allocation. Power losses in straight-ahead driving and a ramp steer maneuver for different motor types and under different wheel torque allocation schemes are analyzed in an offline simulation approach. Significant reductions in motor power losses are achieved for two motor types using an optimization scheme based on look-up tables of motor loss data. Energy loss minimization cannot be achieved through a direct quadratic approximation of the power losses.
Electric vehicles (EVs) with four individually controlled drivetrains are over-actuated systems, and therefore, the total wheel torque and yaw moment demands can be realized through an infinite number of feasible wheel torque combinations. Hence, an energy-efficient torque distribution among the four drivetrains is crucial for reducing the drivetrain power losses and extending driving range. In this paper, the optimal torque distribution is formulated as the solution of a parametric optimization problem, depending on the vehicle speed. An analytical solution is provided for the case of equal drivetrains, under the experimentally confirmed hypothesis that the drivetrain power losses are strictly monotonically increasing with the torque demand. The easily implementable and computationally fast wheel torque distribution algorithm is validated by simulations and experiments on an EV demonstrator, along driving cycles and cornering maneuvers. The results show considerable energy savings compared to alternative torque distribution strategies.
Electric vehicles with multiple motors permit continuous direct yaw moment control, also called torque-vectoring. This allows to significantly enhance the cornering response, e.g., by extending the linear region of the vehicle understeer characteristic, and by increasing the maximum achievable lateral acceleration. These benefits are well documented for human-driven cars, yet limited information is available for autonomous/driverless vehicles. In particular, over the last few years, steering controllers for automated driving at the cornering limit have considerably advanced, but it is unclear how these controllers should be integrated alongside a torque-vectoring system. This contribution discusses the integration of torque-vectoring control and automated driving, including the design and implementation of the torque-vectoring controller of an autonomous electric vehicle for a novel racing competition. The paper presents the main vehicle characteristics and control architecture. A quasi-static model is introduced to predict the understeer characteristics at different longitudinal accelerations. The model is coupled with an off-line optimization for the a-priori investigation of the potential benefits of torque-vectoring. The systematic computation of the achievable cornering limits is used to specify and design realistic maps of the reference yaw rate, and a non-linear feedforward yaw moment contribution providing the reference cornering response in quasi-static conditions. A gain scheduled proportional integral controller increases yaw damping, thus enhancing the transient response. Simulation results demonstrate the effectiveness of the proposed approach.
In electric vehicles with multiple motors, the individual wheel torque control, i.e., the so-called torque-vectoring, significantly enhances the cornering response and active safety. Torque-vectoring can also increase energy efficiency, through the appropriate design of the reference understeer characteristic and the calculation of the wheel torque distribution providing the desired total wheel torque and direct yaw moment. To meet the industrial requirements for real vehicle implementation, the energy-efficiency benefits of torque-vectoring should be achieved via controllers characterised by predictable behaviour, ease of tuning and low computational requirements. This paper discusses a novel energy-efficient torque-vectoring algorithm for an electric vehicle with in-wheel motors, which is based on a set of rules deriving from the combined consideration of: i) the experimentally measured electric powertrain efficiency maps; ii) a set of optimisation results from a non-linear quasi-static vehicle model, including the computation of tyre slip power losses; and iii) drivability requirements for comfortable and safe cornering response. With respect to the same electric vehicle with even wheel torque distribution, the simulation results, based on an experimentally validated vehicle dynamics simulation model, show: a) up to 4% power consumption reduction during straight line operation at constant speed; b) >5% average input power saving in steady-state cornering at lateral accelerations >3.5 m/s2; and c) effective compensation of the yaw rate and sideslip angle oscillations during extreme transient tests.
Thanks to their road safety potential, automated vehicles are rapidly becoming a reality. In the last decade, automated driving has been the focus of intensive automotive engineering research, with the support of industry and governmental organisations. In automated driving systems, the path tracking layer defines the actuator commands to follow the reference path and speed profile. Model predictive control (MPC) is widely used for trajectory tracking because of its capability of managing multi-variable problems, and systematically considering constraints on states and control actions, as well as accounting for the expected future behaviour of the system. Despite the very large number of publications of the last few years, the literature lacks a comprehensive and updated survey on MPC for path tracking. To cover the gap, this literature review deals with the research conducted from 2015 until 2021 on model predictive path tracking control. Firstly, the survey highlights the significance of MPC in the recent path tracking control literature, with respect to alternative control structures. After classifying the different typologies of MPC for path tracking control, the adopted prediction models are critically analysed, together with typical optimal control problem formulations. This is followed by a summary of the most relevant results, which provides practical design indications, e.g., in terms of selection of prediction and control horizons. Finally, the most recent development trends are analysed, together with likely areas of further investigations, and the main conclusions are drawn.
Copyright © 2015 SAE International.This paper discusses the torque-fill capability of a novel hybrid electric drivetrain for a high-performance passenger car, originally equipped with a dual-clutch transmission system, driven by an internal combustion engine. The paper presents the simulation models of the two drivetrains, including examples of experimental validation during upshifts. An important functionality of the electric motor drive within the novel drivetrain is to provide torque-fill during gearshifts when the vehicle is engine-driven. A gearshift performance indicator is introduced in the paper, and the two drivetrain layouts are assessed in terms of gearshift quality performance for a range of maneuvers.
Direct yaw moment controllers improve vehicle stability and handling in severe manoeuvres. In direct yaw moment control implementations based on Linear Quadratic Regulators (LQRs), the control system performance is limited by the unmodelled dynamics and parameter uncertainties. To guarantee robustness with respect to uncertainties, this paper proposes a gain scheduled Robust Linear Quadratic Regulator (RLQR), in which an extra control term is added to the feedback contribution of a conventional LQR to limit the closed-loop tracking error in a neighbourhood of the origin of its state-space, despite the uncertainties and disturbances acting on the plant. In addition, the intrinsic parameter-varying nature of the vehicle dynamics model with respect to the longitudinal vehicle velocity can compromise the closed-loop performance of fixed-gain controllers in varying driving conditions. Therefore, in this study the control gains optimally vary with velocity to adapt the closed-loop system to the variations of this parameter. The effectiveness of the proposed RLQR in improving the robustness of a classical LQR against model uncertainties and parameter variations is proven analytically, numerically and experimentally. The simulation and vehicle test results are consistent with the formal analysis proving that the RLQR reduces the ultimate bound of the error dynamics.
This paper presents a novel 4-wheel-drive electric vehicle layout consisting of one on-board electric drivetrain per axle. Each drivetrain includes a simplified clutch-less 2-speed transmission system and an open differential, to transmit the torque to the wheels. This drivetrain layout allows eight different gear state combinations at the vehicle level, thus increasing the possibility of running the vehicle in a more energy efficient state for the specific wheel torque demand and speed. Also, to compensate the torque gap during gearshifts, a ‘torque-fill’ controller was developed that varies the motor torque on the axle not involved in the gearshift. Experimental tests show the effectiveness of the developed gearshift strategy extended with the torque-fill capability. Energy efficiency benefits are discussed by comparing the energy consumptions of the case study vehicle controlled through a constant front-to-total wheel torque distribution and conventional gearshift maps, and the same vehicle with an energy management system based on an off-line optimization. Results demonstrate that the more advanced controller brings a significant reduction of the energy consumption at constant speed and along different driving cycles.
Electric vehicles with multiple motors permit continuous direct yaw moment control, also called torque-vectoring. This allows to significantly enhance the cornering response, e.g., by extending the linear region of the vehicle understeer characteristic, and by increasing the maximum achievable lateral acceleration. These benefits are well documented for human-driven cars, yet limited information is available for autonomous/driverless vehicles. In particular, over the last few years, steering controllers for automated driving at the cornering limit have considerably advanced, but it is unclear how these controllers should be integrated alongside a torque-vectoring system. This contribution discusses the integration of torque-vectoring control and automated driving, including the design and implementation of the torque-vectoring controller of an autonomous electric vehicle for a novel racing competition. The paper presents the main vehicle characteristics and control architecture. A quasi-static model is introduced to predict the understeer characteristics at different longitudinal accelerations. The model is coupled with an off-line optimization for the a-priori investigation of the potential benefits of torque-vectoring. The systematic computation of the achievable cornering limits is used to specify and design realistic maps of the reference yaw rate, and a non-linear feedforward yaw moment contribution providing the reference cornering response in quasi-static conditions. A gain scheduled proportional integral controller increases yaw damping, thus enhancing the transient response. Simulation results demonstrate the effectiveness of the proposed approach.
This paper presents comparative analyses of steering wheel responses of various layouts for heavy goods vehicles, including rigid and articulated configurations. Newton and Lagrange techniques have been adopted to formulate and verify the generalized linear model for multi-axle rigid and articulated vehicles. The model is then used to simulate, analyze and compare steering angle response of a variety of commercially available vehicles. The study includes the analyses of steady state response and dynamic behavior for different layouts in terms of axle positions, number of axles and multiple steered axles.
Nonlinear model predictive control is proposed in multiple academic studies as an ad-vanced control system technology for vehicle operation at the limits of handling, allow-ing high tracking performance and formal consideration of system constraints. How-ever, the implementation of implicit nonlinear model predictive control (NMPC), in which the control problem is solved on-line, poses significant challenges in terms of computational load. This issue can be overcome through explicit NMPC, in which the optimization problem is solved off-line, and the resulting explicit solution, with guar-anteed level of sub-optimality, is evaluated on-line. Due to the simplicity of the explicit solution, the real-time execution of the controller is possible even on automotive control hardware platforms with low specifications. The explicit nature of the control law fa-cilitates feasibility checks and functional safety validation. This study presents a yaw and lateral stability controller based on explicit NMPC, actuated through the electro-hydraulically controlled friction brakes of the vehicle. The controller performance is demonstrated during sine-with-dwell tests simulated with a high-fidelity model. The analysis includes a comparison of implicit and explicit implementations of the control system.
In automated driving system architectures (see the classification according to [1]), three layers can be typically defined [2]: (i) The perception layer, aimed at detecting the conditions of the environment surrounding the vehicle, e.g., by identifying the appropriate lane and the presence of obstacles on the track; (ii) The reference generation layer, providing the reference signals, e.g., in the form of the reference trajectory to be followed by the vehicle, based on the inputs from the perception layer; (iii) The control layer, defining the commands required for ensuring the tracking performance of the reference trajectory. These commands are usually expressed in terms of reference steering angles (usually on the front axle only) and traction/braking torques. This chapter focuses on the control layer and, in particular, the steering control for autonomous driving, also defined as path tracking control. The foundations of path tracking control for autonomous driving date back to well-known theoretical and experimental studies on robotic systems and driver modeling, detailed in several papers and textbooks (e.g., see the driver model descriptions in [3–9]). Moreover, automated driving experiments with different controllers have been conducted since the 1950s and 1960s, by using inductive cables or magnetic markers embedded in roadways to indicate the reference path [10, 11]. This contribution presents a survey of the main control techniques and formulations adopted to ensure that the automatically driven vehicles follow the reference trajectory, including analysis of extreme maneuvering conditions. The discussion will be based on a selection of different control structures, at increasing levels of complexity and performance. The focus will be on whether complex steering controllers are actually beneficial to autonomous driving. This is an important point, considering that Stanley and Sandstorm, the vehicles that obtained the first two places at the DARPA Grand Challenge (2004–2005), used very simple steering control laws based on kinematic vehicle models. In contrast to this, Boss, the autonomous vehicle winning the DARPA Urban Challenge (2007), was characterized by a far more advanced model predictive control strategy [12–15]. The main formulas for the different steering control structures will be concisely provided as a tutorial on the control system implementations, so that the reader can actually appreciate the characteristics of each formulation, and ultimately refer to the original papers in the case of specific interest. Also, the main simulation and experimental results obtained through the implementation of each control structure will be reported and critically analyzed. The chapter is organized as follows: • Section 5.2 presents path tracking methods based on simple geometric relationships, and a chained controller relying on a vehicle kinematic model, i.e., developed under the approximation of zero slip angles on the front and rear tires. • The first part of Sect. 5.3 deals with conventional feedback controllers designed with a simplified dynamic model of the vehicle system, i.e., the well-known linear single-track vehiclemodel. The second half of Sect. 5.3 discusses relatively simple optimal control formulations, e.g., linear quadratic regulators, without and with feedforward contributions, and including the concept of preview in their most advanced declination. The layout of Sects. 5.2 and 5.3 mostly follows the guideline of a very relevant previous survey work [16], dating back to 2009, which critically assessed path tracking control methods through vehicle simulations with the software package CarSim. • Section 5.4 discusses a couple of sliding mode formulations, one of them based on the important concept of center of percussion, and briefly mentions other examples of path tracking controllers, e.g., based on H1 control and backstepping control. • Section 5.5 presents in detail the latest developments in the subject area, through a selection of examples of advanced controllers (i.e., path tracking controllers for autonomous racing and model predictive controllers) from recently published papers, including critical analysis of their specific benefits. • Section 5.6 provides concluding remarks and ideas for future research on the subject.
This study applies nonlinear model predictive control (NMPC) to the torque-vectoring (TV) and front-to-total anti-roll moment distribution control of a four-wheel-drive electric vehicle with in-wheel-motors, a brake-by-wire system, and active suspension actuators. The NMPC cost function formulation is based on energy efficiency criteria, and strives to minimize the power losses caused by the longitudinal and lateral tire slips, friction brakes, and electric powertrains, while enhancing the vehicle cornering response in steady-state and transient conditions. The controller is assessed through simulations using an experimentally validated high-fidelity vehicle model, along ramp steer and multiple step steer maneuvers, including and excluding the direct yaw moment and active anti-roll moment distribution actuations. The results show: 1) the substantial enhancement of energy saving and vehicle stabilization performance brought by the integration of the active suspension contribution and TV; 2) the significance of the power loss terms of the NMPC formulation on the results; and 3) the effectiveness of the NMPC with respect to the benchmarking feedback and rule based controllers.
This article deals with a novel 2-speed transmission system specifically designed for electric axle applications. The design of this transmission permits seamless gearshifts and is characterized by a simple mechanical layout. The equations governing the overall system dynamics are presented in the paper. The principles of the control system for the seamless gearshifts achievable by the novel transmission prototype - currently under experimental testing at the University of Surrey and on a prototype vehicle - are analytically demonstrated and detailed through advanced simulation tools. The simulation results and sensitivity analyses for the main parameters affecting the overall system dynamics are presented and discussed. © 2011 SAE International.
Electric vehicles with individually controlled drivetrains allow torque-vectoring, which improves vehicle safety and drivability. This paper investigates a new approach to the concurrent control of yaw rate and sideslip angle. The proposed controller is a simple single input single output (SISO) yaw rate controller, in which the reference yaw rate depends on the vehicle handling requirements, and the actual sideslip angle. The sideslip contribution enhances safety, as it provides a corrective action in critical situations, e.g., in case of oversteer during extreme cornering on a low friction surface. The proposed controller is experimentally assessed on an electric vehicle demonstrator, along two maneuvers with quickly variable tire-road friction coefficient. Different longitudinal locations of the sideslip angle used as control variable are compared during the experiments. Results show that: i) the proposed SISO approach provides significant improvements with respect to the vehicle without torque-vectoring, and the controlled vehicle with a reference yaw rate solely based on the handling requirements for high-friction maneuvering; and ii) the control of the rear axle sideslip angle provides better performance than the control of the sideslip angle at the centre of gravity.
Model predictive control (MPC) is increasingly finding its way into industrial applications, due to its superior tracking performance and ability to formally handle system constraints. However, the real-time capability problems related to the conventional implicit model predictive control (i-MPC) framework are well known, especially when targeting low-cost electronic control units (ECUs) for high bandwidth systems, such as automotive active suspensions, which are the topic of this paper. In this context, to overcome the real-time implementation issues of i-MPC, this study proposes explicit model predictive control (e-MPC), which solves the optimization problem off-line, via multi-parametric quadratic programming (mp-QP). e-MPC reduces the on-line algorithm to a function evaluation, which replaces the computationally demanding on-line solution of the quadratic programming (QP) problem. An e-MPC based suspension controller is designed and experimentally validated for a case study Sport Utility Vehicle (SUV), equipped with the active ACOCAR suspension system from the Tenneco Monroe product family. The target is to improve ride comfort in the frequency range of primary ride (< 4 Hz), without affecting the performance at higher frequencies. The proposed e-MPC implementations reduce the root mean square (RMS) value of the sprung mass acceleration by > 40% compared to the passive vehicle set-up for frequencies < 4 Hz, and by up to 19% compared to the same vehicle with a skyhook controller on the 0-100 Hz frequency range.
The front-to-rear wheel torque distribution influences vehicle handling and, ultimately, affects key factors such as vehicle safety and performance. At a glance, as part of the available tire-road friction is used for traction on the driven axle, a Front-Wheel-Drive (FWD) vehicle would be expected to be more understeering than a Rear-Wheel-Drive (RWD) vehicle with equivalent characteristics. However, in specific conditions such effect may be counterbalanced, or even reversed, by the yaw moment caused by the lateral contribution, in the vehicle reference system, of the traction forces at the front wheels. This paper discusses the experimental assessment of the phenomenon in steady-state cornering, for a fully electric vehicle with multiple motors, allowing different front-to-rear wheel torque distributions. The results confirm that the yaw moment effect of the front traction forces is significant, especially at low vehicle speeds and high lateral accelerations. In particular, in the case study maneuvers, the RWD configuration of the vehicle resulted more understeering than the FWD one at the speed of 30 km/h.
This paper presents an H ∞ torque-vectoring control formulation for a fully electric vehicle with four individually controlled electric motor drives. The design of the controller based on loop shaping and a state observer configuration is discussed, considering the effect of actuation dynamics. A gain scheduling of the controller parameters as a function of vehicle speed is implemented. The increased robustness of the H ∞ controller with respect to a Proportional Integral controller is analyzed, including simulations with different tire parameters and vehicle inertial properties. Experimental results on a four-wheel-drive electric vehicle demonstrator with on-board electric drivetrains show that this control formulation does not need a feedforward contribution for providing the required cornering response in steady-state and transient conditions.
Fully electric vehicles with individually controlled drivetrains can provide a high degree of drivability and vehicle safety, all while increasing the cornering limit and the ‘fun-to-drive’ aspect. This paper investigates a new approach on how sideslip control can be integrated into a continuously active yaw rate controller to extend the limit of stable vehicle cornering and to allow sustained high values of sideslip angle. The controllability-related limitations of integrated yaw rate and sideslip control, together with its potential benefits, are discussed through the tools of multi-variable feedback control theory and non-linear phase-plane analysis. Two examples of integrated yaw rate and sideslip control systems are presented and their effectiveness is experimentally evaluated and demonstrated on a four-wheel-drive fully electric vehicle prototype. Results show that the integrated control system allows safe operation at the vehicle cornering limit at a specified sideslip angle independent of the tire-road friction conditions.
Additional publications
Dizqah AM, Lenzo B, Sorniotti A, Gruber P, Fallah S, De Smet J. (2016) 'A Fast and Parametric Torque Distribution Strategy for Four-Wheel-Drive Energy-Efficient Electric Vehicles'. IEEE Transactions on Industrial Electronics, doi: 10.1109/TIE.2016.2540584
De Novellis L, Sorniotti A, Gruber P, Orus J, Rodriguez Fortun J-M, Theunissen J, De Smet J. (2015) 'Direct yaw moment control actuated through electric drivetrains and friction brakes: Theoretical design and experimental assessment'. Mechatronics,doi: 10.1016/j.mechatronics.2014.12.003
Goggia T, Sorniotti A, De Novellis L, Ferrara A, Gruber P, Theunissen J, Steenbeke D, Knauder B, Zehetner J. (2014) 'Integral Sliding Mode for the Torque-Vectoring Control of Fully Electric Vehicles: Theoretical Design and Experimental Assessment'. IEEE Transactions on Vehicular Technology,doi: 10.1109/TVT.2014.2339401
Santucci A., Sorniotti A., Lekakou C. (2014) 'Power Split Strategies for Hybrid Energy Storage Systems for Vehicular Applications'. Journal of Power Sources,doi: 10.1016/j.jpowsour.2014.01.118
De Novellis L., Sorniotti A., Gruber P., Pennycott A. (2014) 'Comparison of Feedback Control Techniques for Torque-Vectoring Control of Fully Electric Vehicles'. IEEE Transactions on Vehicular Technology,doi: 10.1109/TVT.2014.2305475
De Novellis L., Sorniotti A., Gruber P. (2014) 'Wheel Torque Distribution Criteria for Electric Vehicles with Torque-Vectoring Differentials'. IEEE Transactions on Vehicular Technology,doi: 10.1109/TVT.2013.2289371